Table of Contents
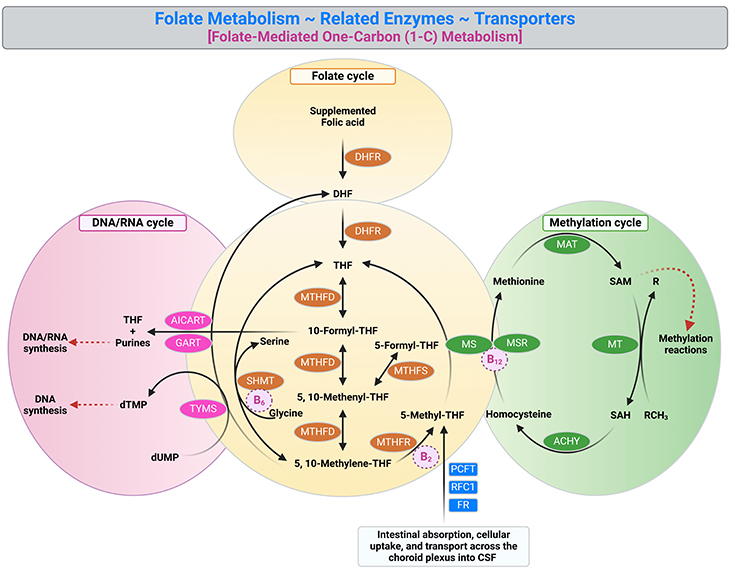
Figure 1. Folate metabolism, related enzymes, and transporters. Folate metabolism (yellow) is at the juncture of two major metabolic cycles: DNA/RNA cycle (pink) and methylation cycle (green). This set of reactions, collectively known as folate-mediated one-carbon (1-C) metabolism, are essential for the production of, for example: (i) thymidine and purine precursors of nucleic acids required for DNA and RNA synthesis and repair; (ii) several amino acids required for protein synthesis, including serine, glycine, and methionine; and (iii) the S-adenosylmethionine (SAM), the universal methylation agent, which an important methyl donor for the methylation of DNA, proteins, as well as neurotransmitters. Various folate derivatives are essential components of cell growth and survival, as a result, changes in folate status can disrupt normal cellular processes. [AHCY, S-adenosylhomocysteine hydrolase; AICART, 5-amino-4-imidazolecarboxamide ribonucleotide transformylase; DHF, dihydrofolate; DHFR, dihydrofolate reductase; dTMP, deoxythymidine monophosphate; dUMP, deoxyuridine monophosphate; FR, folate receptor; GART, β-glycinamide ribonucleotide transformylase; MAT, methionine adenosyl transferase; MS, methionine synthase; MSR, methionine synthase reductase; MT, methionine transferase; MTHFD, methylenetetrahydrofolate dehydrogenase; MTHFR, methylenetetrahydrofolate reductase; MTHFS, methylenetetrahydrofolate synthase; PCFT, proton coupled folate transporter; RFC1, reduced folate carrier 1; SAH, S-adenosylhomocysteine; SAM, S-adenosylmethionine; SHMT, serine hydroxymethyltransferase; THF, tetrahydrofolate; TYMS, thymidylate synthase. [Adapted and modified from: Alam et al., 2020 Trends Pharmacol Sci. May;41(5):349-361.]
Introduction
Folate (vitamin B9) and cobalamin (vitamin B12) are essential for early brain development and function; and play a crucial role in the maintenance of central nervous system (CNS) homeostasis [1]. ‘Homeostasis’ is a self-regulating process by which biological systems maintain stability while adjusting to changing external conditions. Deficiency of folate and/or cobalamin during pregnancy can cause severe malformations in the CNS such as neural tube defects (NTDs).
After birth, folate and/or cobalamin deficiency can cause the following clinical manifestations, for example:
- Anemia
- Failure to thrive
- Recurrent infections
- Psychiatric and neurological symptoms
The folate and the homocysteine metabolic pathways interact at a central step where 5-methyltetrahydrofolate (5-methylTHF) donates its methyl group to homocysteine (Hcy, nonproteinogenic amino acid) to produce methionine (MET, proteinogenic amino acid) and tetrahydrofolate (THF, active form of folate). Methylcobalamin (MeCbl) and folate interact at this critical step.
Both micronutrients have a crucial role in de novo synthesis of DNA or RNA and in biosynthesizing and delivering S-adenosylmethionine (SAM), the universal methyl donor. Until now, severe and mild inherited metabolic disorders (IMD) in folate and cobalamin pathways have been described. The two groups of disorders share some commonalities, nevertheless, differ in the molecular mechanisms, metabolic dysregulation, and disease management.
This blog summarizes selected disorders, including rare and common mutations that govern folate absorption, transport, or dependent enzymes. We will also briefly highlight the clinical significance of folate genetic defects in relation to neurodevelopmental disorders. In the upcoming blog, we will be presenting the clinical implications of cobalamin genetic defects pertaining to neurodevelopmental disorders.
Most importantly, when mutations are discovered early enough, many of the described disorders could be easily treatable by B vitamin supplementation, which often prevents or reverses the clinical manifestation of the disease [2]. Consequently, the screening for mutations is recommended and should be carried out promptly and as early as possible, for example:
- after occurrence of the first symptoms, or
- when a certain constellation of the folate and cobalamin related priority markers are measured, such as elevated total homocysteine (tHcy) and/or methylmalonic acid (MMA).
Genetic Defects in Folate Pathway
(I) Normal Folate Transport and Metabolism
Folate metabolism involves absorption, transport, and intracellular reactions that result in the formation and interconversion of folate coenzymes. (see Figure 1; Table 1-2). Reduced folates act as coenzymes, which serve as one-carbon (1-C) donor or acceptor units in a variety of biochemical reactions, such as (i) methionine synthesis, (ii) histidine catabolism, (iii) purine ring synthesis, (iv) serine-glycine interconversion, and (v) thymidylate synthesis (a key reaction in pyrimidine synthesis). Here is the summary of folate pathway [3]:
- 10-Formyl-tetrahydrofolate (10-formyl-THF) is the folate precursor crucial for purine synthesis.
- 5,10-Methylene-tetrahydrofolate (5,10-Methylene-THF) is folate essential for pyrimidine synthesis.
- Histidine catabolism results in the formation of 5,10-methenyl-tetrahydrofolate(5,10-methenyl-THF), which can subsequently be converted to
either 10-formyl-tetrahydrofolate (10-formyl-THF) or 5,10-methylene-THF. - Serine to glycine conversion requires THF.
- 5,10-Methylene-THF reductase (MTHFR) converts 5,10-methylene-THF to 5-methyl-tetrahydrofolate (5-methyl-THF). This reaction is irreversible in the cells.
- 5-Methyl-THF, is the principle form of folate in extracellular fluids, and serves as the folate transport substrate in the body.
- 5-Methyl-THF transfers its methyl group to methylcobalamin (MeCbl) to generate methionine from homocysteine. This reaction is mediated by the enzyme methionine synthase (MS). The transfer of the methyl group to yield methionine leads to the regeneration of THF.
- Most significantly, when the activity of the MS is impaired, folates become ‘trapped’ in the 5-methyl-THF form because the MTHFR reaction is irreversible in vivo.
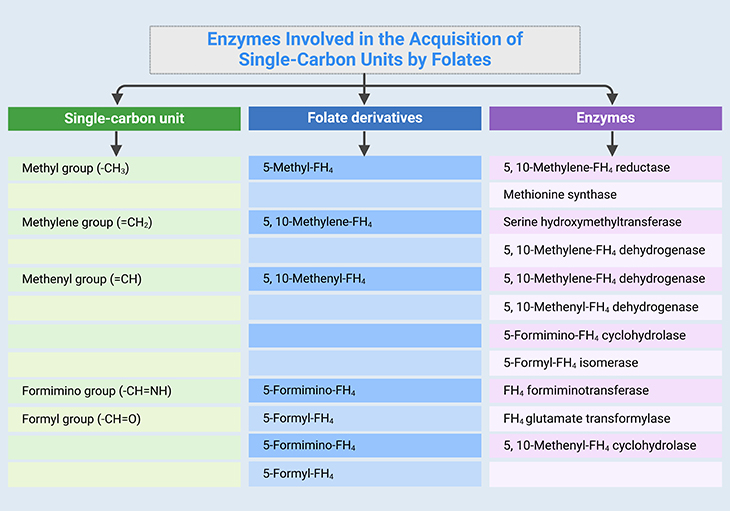
Table 1. Enzymes involved in the acquisition of single-carbon (1-C) units. [FH4, tetrahydrofolate – THF]
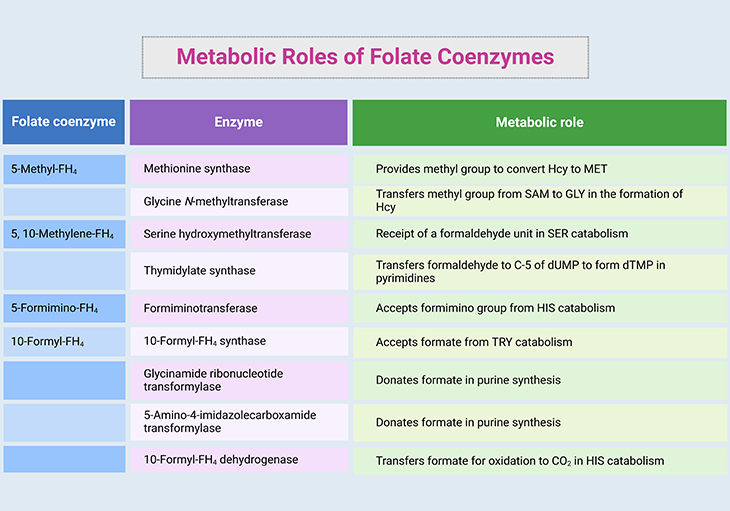
Table 2. Metabolic roles of folate coenzymes. [FH4, tetrahydrofolate – THF; Hcy, homocysteine; MET, methionine; GLY, glycine; HIS, histidine; SER, serine; TRY, tyrosine; SAM, S-adenosylmethionine; dUMP, deoxyuridine monophosphate; dTMP, deoxythymidine monophosphate; C-5, carbon 5; CO2, carbon dioxide]
(II) Folate Transport Pathways
Majority of naturally occurring dietary folates contain a polyglutamate tail that must be hydrolyzed into monoglutamate form prior to gastrointestinal absorption and transport. Dietary folates are metabolized into tetrahydrofolate (THF) derivatives during transit through the intestinal mucosa (in rodents) and/or the liver (in humans). Folates in the liver are either, for example:
- stored in polyglutamate forms,
- secreted into the bile, or
- enter the systemic circulation for tissue distribution.
5-methyl THF is the predominant form of folate that circulates in the blood. Unbound circulating folates are delivered to the kidney for reabsorption. Folates are anionic at physiological pH; they cannot cross biological membranes through diffusion and must depend on specific transport systems for their permeability into the cells and across various epithelia. Thus far, three major folate transport pathways have been characterized in human tissues:
- proton-coupled folate transporter (PCFT),
- reduced folate carrier (RFC), and
- folate receptors (FRs).
Proposed cellular localization of folate transport pathways in select tissues is illustrated and described in more detail in the following blogs. (cf. previous blogs entitled as: (i) ‘Folate Transport Systems – I: Transmembrane Carriers,’ (ii) ‘Folate Transport Systems – II: Folate Receptors,’ (iii) ‘Intestinal Absorption of Dietary Folates,’ (iv) ‘Folate Homeostasis and Tissue Accumulation: What You Need to Know. ’ ).
Inherited Disorders of Folate Transport and Metabolism
Several inherited disorders of folate metabolism and transport have been described [4] (see Table 2), for example:
- MTHFR deficiency,
- MTR deficiency (either caused by mutations in MTR gene or MTRR gene),
- ecerebral folate deficiency (CFD) (caused by FOLR1 mutation),
- hereditary folate malabsorption, and
- glutamate formiminotransferase (FTCD) deficiency.
Besides, several putative inherited disorders related to folate metabolism are described in the literature, for instance:
- DHFR deficiency,
- cellular uptake defects, and
- 5,10-methyleneTHF cyclohydrolase deficiency (part of the trifunctional enzyme 5,10-methyleneTHF dehydrogenase 1 – MTHFD1).
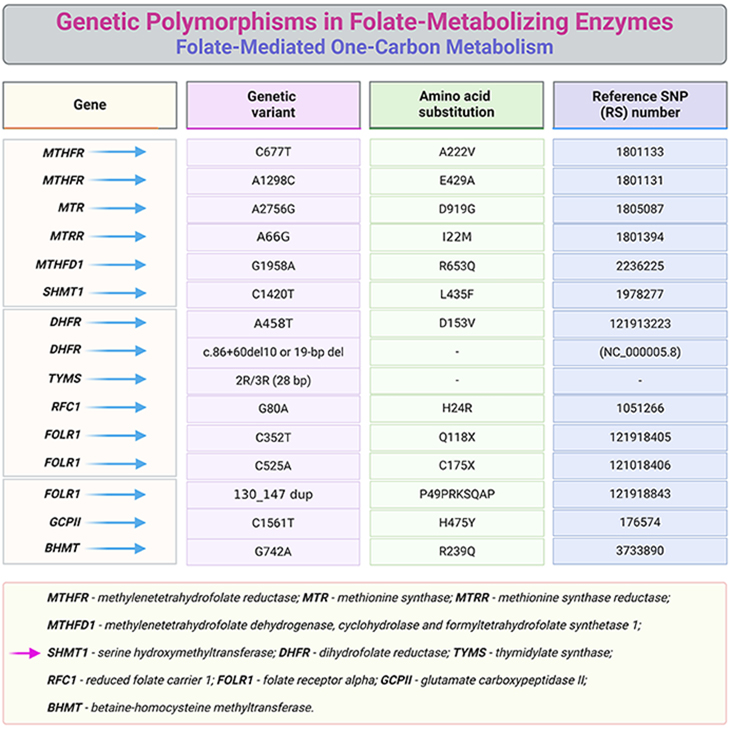
Table 3. Common genetic polymorphisms in folate-metabolizing enzymes.
Neurological Consequences of Folate Deficiency
Defective or impaired folate transport is associated with various types of neurological and neurodevelopmental complications. Here we will briefly discuss five neurological disorders that have been linked to genetic defects in folate pathways and consequently to folate deficiency.
- Hereditary folate malabsorption
- Cerebral folate deficiency (CFD)
- Neural tube defects (NTDs)
- Kearns-Sayre syndrome
- Autism spectrum disorders (ASDs)
(1) Hereditary folate malabsorption
Folate deficiency in the CNS, denoted by low-levels of cerebrospinal fluid (CSF) 5-methyl-THF concentrations, has been implicated in disorders involving defects in folate transport systems. Hereditary folate malabsorption is caused by impaired folate transport across various epithelia such as the intestine and choroid plexus, resulting from the mutations in the SLC46A1 gene [5-6]. It manifests a few months after birth, causing affected newborns to develop, for example:
- severe folate deficiency with megaloblastic anemia,
- diarrhea,
- oral mucositis (stomatitis), and
- recurrent infections due to immune deficiency.
Further symptoms include, for instance:
- poor feeding,
- failure to thrive, and
- neurological manifestations including seizures and developmental delays; peripheral neuropathies, motor impairment, ataxia, and mental/cognitive impairment.
- If left untreated, patients might experience recurrent seizures.
This disorder has been reported in about 30 patients and mostly females are affected. Treatment for this disorder involves parenteral administration of 5-formyl-THF (folinic acid). While this treatment approach is sufficient to correct for peripheral symptoms such as anemia, the accompanying neurological defects are not easily addressed since patients require supraphysiological folate concentrations in blood to be able to increase CSF folate. As a result, increasing folate permeability into the brain or finding alternative routes for brain folate transport can lead to significant therapeutic benefits.
(2) Cerebral folate deficiency (CFD)
Cerebral folate deficiency (CFD) is a neurological syndrome caused by, for example:
- inactivating mutations in FOLR1 gene, or
- the generation of autoantibodies against FRα.
The disease is characterized by impaired folate transport across the choroid plexus, but not in the intestine [7]. Therefore, when dietary folate intake is sufficient, patients show normal concentrations of serum folate, but very low CSF 5-methyl-THF (<5 nM). Patients appear normal at birth and throughout infancy and only exhibit the following neurological symptoms at 2 – 3 years of age, for example:
-
- abnormal brain myelination,
- psychomotor regression,
- epilepsy, and
- ataxia.
The late onset of CFD compared with hereditary folate malabsorption could be due to the presence of normal blood folate levels, which allow for folate transport at the blood-brain barrier (BBB). However, in hereditary folate malabsorption, the presence of severe folate deficiency may limit the protective effect of the BBB.
CFD is a very rare disorder with only 13 confirmed cases hitherto. FRα is the most abundant folate transporter in the choroid plexus, supporting its role as major folate transporter across the blood-CSF barrier (BCSFB). It should be noted that despite normal folate uptake across the BBB, loss of FRα activity in patients with CFD ultimately leads to neurological deficits. These results indicate that there may be insufficient folate transport at the BBB to sustain normal brain development.
Normalizing CSF folate in children affected with CFD or those with hereditary folate malabsorption can be better achieved by administration of high doses of folinic acid or methyl-folate. Oral treatment is less effective than intramuscular injections. Using folic acid is not desirable, because it must be reduced first and the unmetabolized folic acid (UFA) can bind to FRα with a high affinity thus preventing the binding of 5-methylTHF. In addition, due to variability in patient response there is a need for more standardized optimal therapy.
For some, administration of high oral doses of 5-formyl-THF result in increased CSF 5-methyl-THF concentrations and dramatic improvements in neurological outcomes, including:
- normalization of appearance and speech,
- improved motor skills, and
- reduced frequency of epileptic seizures.
In other cases, treatment response is either minimal or requires a substantial amount of time to take effect, for example, 3 years to reverse cortical white matter changes.
Other conditions with neurological manifestations that are associated with CFD are, for instance:
- Schizophrenia,
- Rett syndrome,
- Kearns-Sayre syndrome,
- Aicardi-Goutiere’s syndrome,
- Dihydropteridine reductase deficiency, and
- Hypomyelination with atrophy of the basal ganglion syndrome.
(3) Neural tube defects (NTDs)
Suboptimal folate status in women of childbearing age has been associated to NTD-affected pregnancies [8]. NTDs are severe congenital malformations of the brain and spinal cord caused by the incomplete closure of the neural tube between the days 21-28 post-conception. Defects have two major forms, viz., spinal bifida and anencephaly. Evidence suggests that periconceptional supplementation with folic acid can reduce the incidence of these birth defects.
NTDs occur in >300,000 cases a year where over 95% of them are first occurrence. It has been suggested that folate deficiency was casually related to NTDs. Later studies confirmed that the supplementation with folic acid (FA) alone or in combination with other vitamins reduced the recurrence of NTD pregnancies. Consequently, Centre for Disease Control and Prevention (CDC, Atlanta) recommended:
- A daily oral intake of 400 μg of folic acid (FA) for all women of childbearing age who are capable of becoming pregnant to reduce the risk of having a pregnancy affected by NTDs.
- For women who already have a NTD affected child, the recommendations are 4000 μg/day FA for the prevention of the second occurrence.
In the light these findings, mandatory fortification of white wheat flour and grain products was implemented in the United States and Canda. Fortification is the process of increasing the content of nutrients, which are normally present within a food vehicle (e.g., grain flour, enriched cereal grain products, rice, salt, milk, and margarine).
- FA fortification policies resulted in a reduction of prevalence rate of NTDs, an improvement of the folate concentration in blood, and a reduction of total homocysteine (tHcy) concentrations.
- Women planning a pregnancy were further advised to consume multivitamin supplements containing different doses of folic acid (400 – 5000 μg/day), depending on their NTD risk, for at least three months before conception and throughout the first trimester of pregnancy, which is in addition to the consumption of folate rich foods.
The research concerning the underlying mechanism of the preventions of NTDs by FA currently focus on, for example:
- the abnormal folate metabolism,
- the Hcy metabolism [plasma and amniotic fluid (tHcy) is higher in NTD infants and their mothers], or
- variations in folate-related enzymes.
(4) Kearns-Sayre syndrome
Kearns-Sayre syndrome is a rare neuromuscular disorder caused by deletions in mitochondrial DNA [9]. Initial reports indicated an association between Kearns-Sayre syndrome and folate deficiency. In particular, female patients revealed low plasma and CSF folate levels. Further observations established that individuals with Kearns-Sayre syndrome had low total folate and 5-methyl-THF levels in their CSF. Remarkably, it was further reported that choroid plexus epithelial cells collected from these patients displayed an enlarged and granular phenotype, which may presumably cause the failure of folate transport into the CSF.
This disorder is primarily characterized by the following clinical features, for example:
- chronic progressive external ophthalmoplegia with drooping and paralysis of the eyelids, and
- pigmentary retinopathy, leading to mild impaired vision.
As the disease progresses, other associated symptoms develop, such as:
- cardiac conduction disorders,
- muscle weakness,
- endocrine disorders,
- neurologic dysfunction, and
- cerebral folate deficiency.
(5) Autism spectrum disorders (ASDs)
Numerous lines of evidence suggest that periconceptional folic acid intake reduces the risk of ASD by ≈ 40%. Although these reports indicate a potential association between maternal folate status and ASD, the exact mechanism pertaining to the protective role of folate remains largely unknown [10]. Over recent years, low CSF folate was observed in children with ADS, particular Rett syndrome and infantile low-function autism. These autistic individuals are present with FRα autoantibodies, which block folate binding to FRα or disrupt receptor function. Frye and colleagues reported a high prevalence (50 – 70%) of FRα autoantibodies in serum of autistic patients compared with control [10-11]. Moreover:
- Human trials involving high oral dosing of folinic acid (5-formyl-THF) have also shown partial recovery of neurological and social impairments in patients with infantile autism.
- Marked improvements in attention, language, and stereotypical behavior was further reported in over 60% of patients treated with 5-formyl-THF (2 mg/kg/day) compared with nontreated controls.
- In a 12-week randomized doble-blind placebo-controlled trial, 5-formyl-THF supplementation (2mg/kg/day) significantly improved verbal communication of patients expressing FRα autoantibodies.
Despite the promising effects of folate intervention in ASD, there is yet a need to establish more effective therapies. As indicated in these trials, prolonged consumption of 5-formyl-THF at such high doses could result in several adverse events, such as:
-
- insomnia,
- gastrointestinal reflux, and
- worsening aggression.
Considering the fact that autism is a lifelong disorder, finding an optimal therapeutic approach with limited adverse effects is of paramount importance.
Take Home Messages
- Folate transport in human brain is mediated by three major pathways: proton-coupled folate transporter ( PCFT; SLC46A1 ), reduced folate carrier ( RFC1; SLC19A1 ); and folate receptor alpha ( FRα, FOLR1 ).
- Folate delivery to the brain primarily occurs at the choroid plexus (i.e., blood-CSF barrier) through FRα and PCFT.
- Genetic defects in folate pathway, i.e., loss-of-function mutations in these three transport systems have been implicated in a number of childhood neurological disorders, including hereditary folate malabsorption, cerebral folate deficiency (CFD), and autism spectrum disorders (ASDs).
- At present, there is limited knowledge on the contribution of other brain regions (i.e., blood-brain barrier, BBB) to overall brain folate uptake.
- Enhancing folate permeability into the brain or finding alternative routes for effective brain folate transport could lead to significant therapeutic benefits.
Summary and Conclusions
In this blog, we have briefly highlighted the role of genetic defects in folate metabolism and its impact on brain function. As well as the clinical significance of cerebral folate transport in relation to neurodevelopmental disorders associated with folate deficiency.
Disorders in the folate transport and/or metabolism that affect folate absorption, transport, or dependent enzymes, cause severe neurological symptoms that are in some situations reversible after supplementing the co-enzymes form of the vitamin. Severe elevation in plasma or urine concentrations of total homocysteine (tHcy) and/or methylmalonic acid (MMA) (priority folate/cobalamin biomarkers) can be considered as a screening test for these disorders. However, it is crucial to identify the defective pathway in order to supplement the appropriate form of the vitamin as early as possible. The neurological and neurodevelopmental complications in patients with defects in the folate related reactions underline the importance of the vitamins in the pre- and post-natal development of the CNS.
In a nutshell, micronutrient such as folates are essential for the brain development and function. Abnormalities in brain folate transport is linked to a number of neurodevelopmental conditions and current therapeutic approaches are not sufficiently effective. Existing treatment strategies involve administration of high doses of 5-formyl-THF (folinic acid) in order to deliver sufficient folate to the brain to sustain neural development. Despite achieving high blood folate levels, CSF folate concentration can remain below the normal range and neurological signs, specifically seizures, are difficult to control. Thus, taken together, identifying novel strategies for enhancing folate permeability to the CNS is of paramount importance!
For information on autism monitoring, screening and testing please read our blog.
References
- Black MM. Effects of vitamin B12 and folate deficiency on brain development in children. Food Nutr Bull. 2008 Jun;29(2 Suppl):S126-31. doi: 10.1177/15648265080292S117. PMID: 18709887; PMCID: PMC3137939.
https://pubmed.ncbi.nlm.nih.gov/18709887/ - Reynolds E. Vitamin B12, folic acid, and the nervous system. Lancet Neurol. 2006 Nov;5(11):949-60. doi: 10.1016/S1474-4422(06)70598-1. PMID: 17052662.
https://pubmed.ncbi.nlm.nih.gov/17052662/ - Lyon P, Strippoli V, Fang B, Cimmino L. B Vitamins and One-Carbon Metabolism: Implications in Human Health and Disease. Nutrients. 2020 Sep 19;12(9):2867. doi: 10.3390/nu12092867. PMID: 32961717; PMCID: PMC7551072.
https://pubmed.ncbi.nlm.nih.gov/32961717/ - Fowler B. Genetic defects of folate and cobalamin metabolism. Eur J Pediatr. 1998 Apr;157 Suppl 2:S60-6. doi: 10.1007/pl00014306. PMID: 9587028.
https://pubmed.ncbi.nlm.nih.gov/9587028/ - Zhao R, Aluri S, Goldman ID. The proton-coupled folate transporter (PCFT-SLC46A1) and the syndrome of systemic and cerebral folate deficiency of infancy: Hereditary folate malabsorption. Mol Aspects Med. 2017 Feb;53:57-72. doi: 10.1016/j.mam.2016.09.002. Epub 2016 Sep 21. PMID: 27664775; PMCID: PMC5253092.
https://pubmed.ncbi.nlm.nih.gov/27664775/ - Aluri S, Zhao R, Lubout C, Goorden SMI, Fiser A, Goldman ID. Hereditary folate malabsorption due to a mutation in the external gate of the proton-coupled folate transporter SLC46A1. Blood Adv. 2018 Jan 5;2(1):61-68. doi: 10.1182/bloodadvances.2017012690. PMID: 29344585; PMCID: PMC5761628.
https://pubmed.ncbi.nlm.nih.gov/29344585/ - Pope S, Artuch R, Heales S, Rahman S. Cerebral folate deficiency: Analytical tests and differential diagnosis. J Inherit Metab Dis. 2019 Jul;42(4):655-672. doi: 10.1002/jimd.12092. Epub 2019 May 2. PMID: 30916789.
https://pubmed.ncbi.nlm.nih.gov/30916789/ - Pitkin RM. Folate and neural tube defects. Am J Clin Nutr. 2007 Jan;85(1):285S-288S. doi: 10.1093/ajcn/85.1.285S. PMID: 17209211.
https://pubmed.ncbi.nlm.nih.gov/17209211/ - Shemesh A, Margolin E. Kearns-Sayre Syndrome. In: StatPearls [Internet]. Treasure Island (FL): StatPearls Publishing; 2024 Jan.2023 Jul 17. PMID: 29493966 Bookshelf ID: NBK482341.
https://www.ncbi.nlm.nih.gov/books/NBK482341/ - Frye RE, Delhey L, Slattery J, Tippett M, Wynne R, Rose S, Kahler SG, Bennuri SC, Melnyk S, Sequeira JM, Quadros E. Blocking and Binding Folate Receptor Alpha Autoantibodies Identify Novel Autism Spectrum Disorder Subgroups. Front Neurosci. 2016 Mar 9;10:80. doi: 10.3389/fnins.2016.00080. PMID: 27013943; PMCID: PMC4783401.
https://pubmed.ncbi.nlm.nih.gov/27013943/ - Frye RE, Slattery J, Delhey L, Furgerson B, Strickland T, Tippett M, Sailey A, Wynne R, Rose S, Melnyk S, Jill James S, Sequeira JM, Quadros EV. Folinic acid improves verbal communication in children with autism and language impairment: a randomized double-blind placebo-controlled trial. Mol Psychiatry. 2018 Feb;23(2):247-256. doi: 10.1038/mp.2016.168. Epub 2016 Oct 18. PMID: 27752075; PMCID: PMC5794882.
https://pubmed.ncbi.nlm.nih.gov/27752075/