Table of Contents
- Introduction
- Folate Receptors (FRs)
- Folate Receptor – Alpha (FOLR1, FRα)
- Folate Receptor – Beta (FOLR2, FRβ)
- Folate Receptor – Gamma (FOLR3, FRγ)
- Folate Receptor-Mediated Endocytosis
- Genetic Deletions of Folate Receptors
- Folate Transport Across the Blood-Brain Barrier (BBB) and the Blood-CSF Barrier
- Folate Transport Across the Placenta
- Clinical Significance
- Conclusion
- Did You Know About Folate Receptor Autoantibodies (FRAAs) and Brain Development?
- References
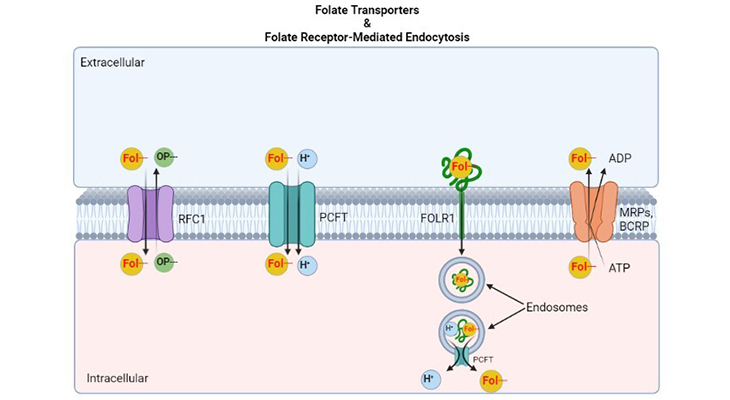
Figure 1. Major Folate Transporters – the processes that regulate transmembrane folate flows and gradients. Folate transporters. (a) The reduced folate carrier (RFC1; SLC19A1) is an anion exchanger that utilizes the transmembrane organic phosphate (OP–) gradient to achieve uphill transport into cells. (b) The proton coupled folate transporter (PCFT; SLC46A1) is a folate (Fol-)-H+ symporter that functions most efficiently in an acidic extracellular environment. (c) Folate receptors, FOLR1 (FRα) and FOLR2 (FRβ) are high-affinity folate-binding proteins that transport folates into cells via endocytotic mechanisms. Once in the cytoplasm, the vesicle (endosome) acidifies, and folate is dissociated from the receptor, and is exported from cells at least in part by PCFT. (d) The multidrug resistance associated proteins (MRPs; ABCC1-ABCC5) and/or breast cancer resistant protein (BCRP; ABCG2), are the ATP binding cassette exporters that can serve as folate exporters from the cells back into the extracellular compartment and thereby counter the inward transport mediated by folate importers.
Introduction
Members of the family of B9 vitamins are commonly known as folates. They are derived exclusively from dietary sources and are key one-carbon donors required for de novo synthesis of nucleotides and methionine. Folates are highly hydrophilic molecules that use several genetically distinct and functionally diverse transport systems to enter tissues and cells, viz., reduced folate carrier (RFC), the proton-coupled transporter (PCFT), and the folate receptors (FRs). Each one of those plays a unique role in mediating folate transport across various types of epithelia and into systemic compartments and tissues [1, 2].
Due to recent advances in our understanding of the mechanism of intestinal folate absorption, it is therefore now possible to piece together how these folate transporters contribute, both individually and collectively, to folate homeostasis in humans. Thus, this brief review focuses on the physiological roles of one of the major classes of folate transporters, viz. the FRs (cf. Folate Transport Systems – I: Transmembrane Carriers).
Multigene Family of Folate Receptors | Gene Symbol | |
---|---|---|
1. | Folate receptor-α, adult | FOLR1 |
2. | Folate receptor-β, fetal | FOLR2 |
3. | Folate receptor-γ | FOLR3 |
Table 1. A Family of high-affinity folate receptors.
Folate Receptors (FRs)
A family of three high-affinity and closely related folate FRs, viz., FOLR1, FOLR2, and FOLR3 (FRα, FRβ, and FRγ), also known as folate-binding proteins, are encoded by three distinct genes located on chromosome 11. FRs are homologous proteins (68%-79% identical amino acid sequences), characterized by disparate patterns of tissue expression. The FRs are glycosylphosphatidylinositol-anchored proteins (GPI-APs) that mediate folate transport.
Folate Receptor – Alpha (FOLR1, FRα)
In normal tissues, the expression pattern of FRα is usually confined to the apical membrane where it would not be in contact with the general circulation [1]. FRα is highly expressed in the following adult and fetal tissues, for example:
- The luminal brush border membrane of proximal tubules of kidney
- Basolateral membrane of choroid plexus and retinal pigmental epithelium
- Lung (alveolar cells), bronchial cells, erythropoietic cells, and submandibular salivary gland
- Breast (acinar cells), uterus, fallopian tubes, ovaries, epididymis, and vas deferens
- Placenta (trophoblastic cells) and Fetal cells
In addition, low levels are detected in thyroid gland, and very low levels may be present in gut mucosal cells. FRα is also overexpressed in various types of tumors cells, specifically,
- Non-mucinous adenocarcinomas of the cervix, uterus, and ovary
- Epithelial-derived tumors, such as breast (triple negative), brain, renal, lung (mesotheliomas), as well as colorectal carcinomas
Folate Receptor – Beta (FOLR2, FRβ)
Unlike FRα, FRβ is expressed mainly in the following tissues, for instance:
- Placenta, spleen, and thymus
- Monocytes (CD34+) and normal myelopoiesis.
- Fetal tissue
Besides, high levels are expressed in certain myelogenous leukemia, such as (i) acute myelogenous leukemic cells and (ii) chronic myogenous leukemic cells [3].
Folate Receptor – Gamma (FOLR3, FRγ)
FRγ is a folate-binding protein that in humans is encoded by the FOLR3 gene and is involved in the uptake of folic acid [4]. It is generally expressed in human tissues but not in mouse or rat tissues and presents as a secretory protein. FRγ primarily is localized in the following tissues, such as:
- Reproductive tissues
- Immune system
- Hematopoietic tissues, such as thymus, spleen, and bone marrow
- Ovarian carcinoma, choriocarcinoma of the testis, and primary peritoneal carcinoma
Originally, FRs were identified as a high-affinity soluble plasma folate-binding protein; and it was also found in milk. Typically, FRs are attached to the plasma membrane of cells via GPI-anchor and can be released from the membrane by a specific enzyme, the so-called phospholipase C.
The FRs show high-affinity for a variety of folates with KdS in the nanomolar range. FRs have the highest affinity for folic acid {Kd 1 – 10 nM}. The affinity of 5-methyl tetrahydrofolate (5-methyl THF) for FRα is slightly lower, and its affinity is reduced almost 50-fold further for FRβ. Methotrexate has relatively poor affinity for these receptor proteins [1]. Hence, to summarize:
- Folate receptor has high-affinities for reduced folates in the physiological plasma folate concentration range {(6 to 20 ng/mL or 13.5 to 45.3 nmol/L) – *Folic acid conversion factor: 1ng/mL = 2.265 nmol/L}.
- Transport through this receptor would be somewhat responsive to increased plasma folate levels but would saturate when high doses of folate are given (i.e., supraphysiological levels).
- Folic acid has very high-affinity for this receptor, and folic acid transport would be saturated even at low level of folic acid in plasma.
Folate Receptor-Mediated Endocytosis
Folate transport through the folate-binding protein (FR) occurs through a process called “receptor-mediated endocytosis.” Mechanistically, the folate receptor-folate complex has been shown to internalize via a non-clathrin-mediated classical endocytotic pathway not involving lysosomes [5, 6]. Folate is released from the vesicles (or endosomes) into the cytosol, and the receptor protein rapidly recycles the vesicles back to the plasma membrane (Figure 1). This process is triggered, initially, when:
- A folate molecule binds to a membrane-associated folate receptor on the cell surface.
- This is followed by invagination of the plasma membrane at that site and the formation of a vesicle.
- Next, the formed intact endosome migrates along microtubules in the cytoplasm to the perinuclear endosomal compartment where it is acidified to a pH of ≈ 6.0 – 6.5.
- Eventually, this results in dissociation of the folate from the folate receptor complex.
Finally, the disassociated folate ligand is then exported into the cytoplasm by a further process that requires a “transendosomal pH gradient,” and possibly, this folate export from endosomes is carried out by PCFT.
The physiological roles of folate receptors are unclear in all but a few cases, for instance, the role in kidney folate reabsorption is well established [7]. Even though FRα and FRβ can transport folate into cells, this is a relatively slow process, and inefficient compared with transmembrane transporters such as RFC1. However, it clearly plays an important role in development.
Genetic Deletions of Folate Receptors
It is evident that folate receptors play a significant role in development, loss of both alleles encoding FRα protein is embryonic lethal; and most importantly, the embryos are arrested at the time of neural tube closure, leading to neural tube defects [8]. On the contrary, FRβ null mice are normal, as are mice heterozygous for FRα, i.e., deletion of FRβ genes has no obvious phenotype.
Folate Transport Across the Blood-Brain Barrier (BBB) and the Blood-CSF Barrier
There exist two potential routes of delivery of folates to the brain, viz., (i) the blood-brain barrier (BBB) at the cerebral vascular endothelium; and (ii) the blood-cerebrospinal fluid (CSF) barrier at the choroid plexus.
FRα is neither expressed in brain parenchyma nor is FRα protein evidently detected at the blood-brain barrier. However, RFC1 is localized to the vascular blood-brain barrier, in addition, the PCFT protein is expressed there as well.
The choroid plexus represents the pathway for the active transport of substances and metabolites, including folates, into and out of CSF. It has been demonstrated that FRα plays a critical role in this process and is based on the following observations that [9]:
- The folate-binding protein, FRα, is highly expressed in choroid plexus; and
- Blocking autoantibodies against FRs have been found in the blood of patients with cerebral folate deficiency (CFD).
In this CFD disorder, low levels of CSF folate have consistently been shown even though folate intestinal absorption and folate blood levels are normal. Furthermore, in general, these subjects do not have demonstrable mutations in genes that encode FRα, RFC1, and PCFT.
Folate Transport Across the Placenta
All the three folate transporters, viz., FRβ, RFC1, and PCFT are expressed in the placenta, nevertheless, their exact location and the spatial relationship have not been well established [10]. However, certain animal experiments have evidently shown that FRα plays an important role in the initial phase of folate transport from maternal blood at the apical membrane of the syncytiotrophoblast [11].
Clinical Significance
- Autoantibodies directed against FRα have been linked to neurodevelopmental disorders, most importantly, the following three medical conditions [12]: (i) cerebral folate deficiency syndrome (CFD), (ii) autism spectrum disorders (ASD), and (iii) schizophrenia.
- Recent studies have demonstrated that these neurodevelopmental disorders can effectively be treated with high doses of folinic acid.
- FRα, due to its high expression in some tumor tissues, is a potential and an attractive therapeutic target for the development of various novel anti-cancer agents. Especially, to limit the burden and toxic side-effects on off-target tissues.
- Unlike FRα and FRβ, the expression level of FRγ is correlated more strongly with plasma homocysteine (Hcy). FRγ can metabolize both intracellular and extracellular Hcy, consequently, may decrease plasma Hcy. Thus, overexpression of FRγ may effectively ameliorate Hcy in the blood and reduce Hcy-induced toxicity [13].
Conclusion
In this report, we have elaborated our recent perspectives on one of the major classes of folate transporter, viz., the FRs. FR plays a unique role in mediating folate transport across various epithelia and into systemic compartments and tissues under physiological conditions. Nonetheless, our current understanding of the potential health risks that are associated with an inherited organ-specific folate transport defect that is caused by mutations in FR gene coding for a folate receptor is limited. In addition, dysfunctions due to FR autoantibodies in these FR-mediated transport mechanistic process under pathological conditions is somewhat incomplete; and consequently, its association with various types of disorders, including malignancies as well as neurodevelopmental disorders, such as CFD and its associated neural tube defects (NTDs), ASD, and schizophrenia are gradually evolving.
For information on autism monitoring, screening and testing please read our blog.
References
- Zhao R, Matherly LH, Goldman ID. Membrane transporters and folate homeostasis: intestinal absorption and transport into systemic compartments and tissues. Expert Rev Mol Med. 2009 Jan 28;11:e4. doi: 10.1017/S1462399409000969. PMID: 19173758; PMCID: PMC3770294.
https://pubmed.ncbi.nlm.nih.gov/19173758/ - Visentin M, Diop-Bove N, Zhao R, Goldman ID. The intestinal absorption of folates. Annu Rev Physiol. 2014;76:251-74. doi: 10.1146/annurev-physiol-020911-153251. PMID: 24512081; PMCID: PMC3982215.
https://pubmed.ncbi.nlm.nih.gov/24512081/ - Ross JF, Wang H, Behm FG, Mathew P, Wu M, Booth R, Ratnam M. Folate receptor type beta is a neutrophilic lineage marker and is differentially expressed in myeloid leukemia. Cancer. 1999 Jan 15;85(2):348-57. doi: 10.1002/(sici)1097-0142(19990115)85:2<348::aid-cncr12>3.0.co;2-4. PMID: 10023702.
https://pubmed.ncbi.nlm.nih.gov/10023702/ - Kelemen LE. The role of folate receptor alpha in cancer development, progression and treatment: cause, consequence or innocent bystander? Int J Cancer. 2006 Jul 15;119(2):243-50. doi: 10.1002/ijc.21712. PMID: 16453285.
https://pubmed.ncbi.nlm.nih.gov/16453285/ - Rothberg KG, Ying YS, Kolhouse JF, Kamen BA, Anderson RG. The glycophospholipid-linked folate receptor internalizes folate without entering the clathrin-coated pit endocytic pathway. J Cell Biol. 1990 Mar;110(3):637-49. doi: 10.1083/jcb.110.3.637. PMID: 1968465; PMCID: PMC2116044.
https://pubmed.ncbi.nlm.nih.gov/1968465/
- Kamen BA, Smith AK. A review of folate receptor alpha cycling and 5-methyltetrahydrofolate accumulation with an emphasis on cell models in vitro. Adv Drug Deliv Rev. 2004 Apr 29;56(8):1085-97. doi: 10.1016/j.addr.2004.01.002. PMID: 15094208.
https://pubmed.ncbi.nlm.nih.gov/15094208/
- Birn H, Selhub J, Christensen EI. Internalization and intracellular transport of folate-binding protein in rat kidney proximal tubule. Am J Physiol. 1993 Feb;264(2 Pt 1):C302-10. doi: 10.1152/ajpcell.1993.264.2.C302. PMID: 8447363.
https://pubmed.ncbi.nlm.nih.gov/8447363/
- Piedrahita JA, Oetama B, Bennett GD, van Waes J, Kamen BA, Richardson J, Lacey SW, Anderson RG, Finnell RH. Mice lacking the folic acid-binding protein Folbp1 are defective in early embryonic development. Nat Genet. 1999 Oct;23(2):228-32. doi: 10.1038/13861. PMID: 10508523.
https://pubmed.ncbi.nlm.nih.gov/10508523/
- Ramaekers VT, Rothenberg SP, Sequeira JM, Opladen T, Blau N, Quadros EV, Selhub J. Autoantibodies to folate receptors in the cerebral folate deficiency syndrome. N Engl J Med. 2005 May 12;352(19):1985-91. doi: 10.1056/NEJMoa043160. PMID: 15888699.
https://pubmed.ncbi.nlm.nih.gov/15888699/
- Maddox DM, Manlapat A, Roon P, Prasad P, Ganapathy V, Smith SB. Reduced-folate carrier (RFC) is expressed in placenta and yolk sac, as well as in cells of the developing forebrain, hindbrain, neural tube, craniofacial region, eye, limb buds and heart. BMC Dev Biol. 2003 Jul 29;3:6. doi: 10.1186/1471-213X-3-6. Epub 2003 Jul 29. PMID: 12887734; PMCID: PMC184426.https://pubmed.ncbi.nlm.nih.gov/12887734/
- Sweiry JH, Yudilevich DL. Characterization of folate uptake in guinea pig placenta. Am J Physiol. 1988 Jun;254(6 Pt 1):C735-43. doi: 10.1152/ajpcell.1988.254.6.C735. PMID: 3259839.
https://pubmed.ncbi.nlm.nih.gov/3259839/
- Frye RE, Slattery JC, Quadros EV. Folate metabolism abnormalities in autism: potential biomarkers. Biomark Med. 2017 Aug;11(8):687-699. doi: 10.2217/bmm-2017-0109. Epub 2017 Aug 3. PMID: 28770615.
https://pubmed.ncbi.nlm.nih.gov/28770615/
- Yoshitomi R, Nakayama K, Yamashita S, Kumazoe M, Lin TA, Mei CY, Marugame Y, Fujimura Y, Maeda-Yamamoto M, Kuriyama S, Tachibana H. Plasma Homocysteine Concentration is Associated with the Expression Level of Folate Receptor 3. Sci Rep. 2020 Jun 24;10(1):10283. doi: 10.1038/s41598-020-67288-9. PMID: 32581311; PMCID: PMC7314855
.https://pubmed.ncbi.nlm.nih.gov/32581311/