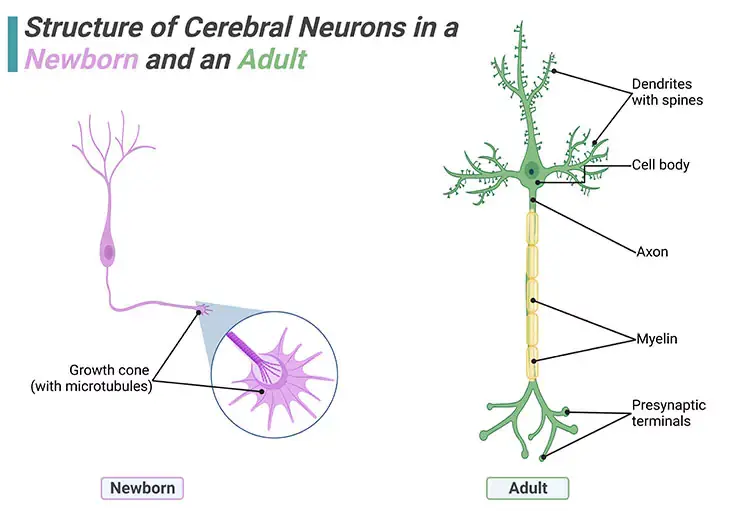
Figure 1. Structure of Cerebral Neurons in a Newborn and an Adult. Individual brain cells undergo enormous elaborations during infancy and early childhood. No new neurons are added postpostnally i.e., after birth, but new dendrites and synapses sprout intensely during a child’s earlier years, causing the cerebral cortex to thicken and its circuitry to grow massively more complex. Brain wiring begins with the outgrowth of axons. Once a newborn neuron has migrated, planting its cell body in a permanent position, it sends out a fine axon shoot with an enlarged tip known as a ‘growth cone.’ At the end of the growth cone are about a dozen long tentacles or projecting spikes that shoot out in all directions and act like radar, picking up all means of navigational signals.
Introduction
Acquiring an understanding of how the brain and mind develop in the first five years of life is the foundation for understanding other key areas of child development, such as sensation, movement, emotion, language, perception, cognition, and “intelligence.” While the study of our biology is important in its own right, it is also tied to development in these other domains and necessary to examine at an early point in our study of children. The lingering question that needs to be addressed is what drives neurotypical brain development? The debate is about nature versus nurture, i.e., how much of a baby’s development is genetically ordained ? – and how much is determined by the environment?
I. Neurotypical Brain Development
The development of the human neurotypical (NT) brain embarks pretty soon after conception and prolongs beyond adolescence into the late twenties or early thirties. Even though a considerable amount of the development takes place within the womb prenatally, i.e., before birth, humans are quite unique in that a great deal of the brain develops postnatally, i.e., after birth. Unlike other animals, changes in brain structure occur throughout the lifespan, even into old age (Figure 1).
(1) Prenatal (Before-Birth) Development
(i) Neural Tube Formation
The brain starts to develop within the first month after conception (i.e., the process of becoming pregnant involving fertilization or implantation or both), with the formation of the neural tube within which the central nervous system (CNS) will eventually grow. This process usually takes about 4 days. The frontal (rostral) part of the tube will form the brain, whereas the rear (caudal) part will form the spinal cord. At first the neural tube will be open at both ends, but it will eventually close. The neural tube is almost completely developed by 3 to 4 weeks after conception [1] (Figure 2).
Most of the brain neurons an individual will have over a lifetime develop during the first 4 months. These neurons develop in the base of the brain and then migrate to other parts of the brain to form specialized structures and systems. Among the earliest neurons to develop are the clusters of cells forming nuclei in the brain stem; as well as a particular type of cell called Purkinje cells that are found in the cerebellar vermis. Neural migration is mostly completed by 20 weeks. Once in position, during the last two months before birth, neurons begin to develop the first axons and dendrites that begin to form the neural networks responsible for information processing (Figure 1).
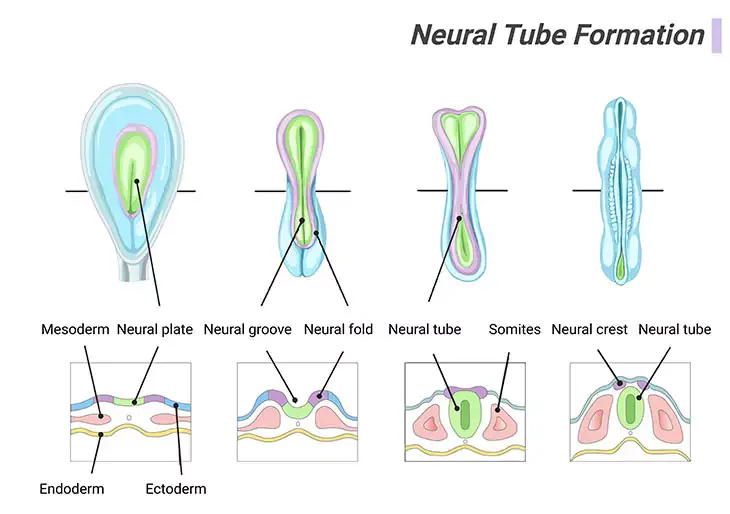
Figure 2. Neural Tube Formation. Apparently, embryonic brain development appears quite rapid. Around twenty-five days (day 25), the neural plate has folded up to form a groove, and then fused along its top edges to form a tube running most of the length of the two-millimeter (2 mm) embryo. By twenty-six days (day 26), this neural tube will have closed, starting in the middle and finishing first in the head end at around twenty-four days postfertilization, and then at the tail end. The closed tube is already enlarged at the top end, where the brain is emerging. The rest of the tube, gradually tapering down toward the tail, will become the spinal cord.
The brain continues to grow rapidly as the baby develops in the womb. The most rapid period of brain growth occurs during the third trimester, when it can grow at the rate of 22 ml per week. Furthermore, recently it has been shown that during the third trimester the cerebellum shows the greatest relative maturation rate of 12.87% per week, exhibiting a volume increase of 384% between 25 to 36 weeks of gestation. By comparison, total brain volume increases by 230% over the same period [2].
(ii) Neurogenesis
Neurogenesis is the process by which nervous system cells, viz., the neurons, are generated by neural stem cells (NSCs) [3]. Briefly, it is brain growth in relation to its organization. Neurogenesis is most intensive during embryonic development and is responsible for creating all the different types of neurons of the organism, however, it continues throughout adult life in a variety of organisms, including humans in a limited fashion. Neurons are terminally differentiated, non-proliferating cells, but they can expand in size, i.e., once generated, neurons do not divide, and many will live the lifespan of the animal, except under extraordinary and usually pathogenic circumstances (Figure 3; Box – 1) [4].
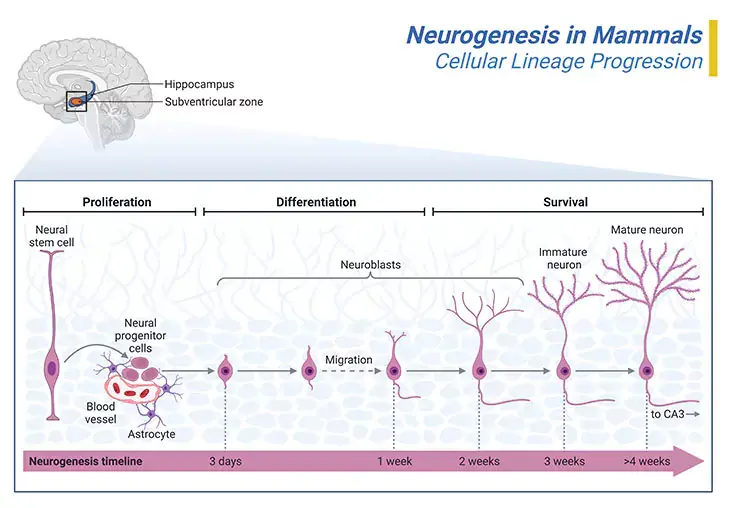
Figure 3. Neurogenesis in Mammals. Neurogenesis initiates in the inner portion of the neural tube in what are called proliferative zone. The most significant of these is the ventricular zone, which is adjacent to the fluid center of the neural tube. The first kind of cells that are produced are called ‘neural progenitor or precursor cells.’ Progenitor cells are very similar to stem cells that are lineage-restricted and/or show limited proliferative capacity. It is within the proliferative zones that the neurons that will form the brain are born. From here they will migrate outwards, often passing through neurons that have already been created. Some cells reach their destination simply by what is called passive migration, i.e., by being pushed outwards by new neurons as they are created in the proliferative zones. The thalamus and parts of the hippocampus are formed in this way. Other neurons will actively migrate, pushing their way through neural cells that have already been generated. The neurons that form the six layers of the cerebral cortex are created by active cell migration. Thus, the neocortex is formed of six layers, numbered I to IV, from the outermost layer I – near to the pia matter, to the inner most layer VI – near to the underling white matter (I – molecular layer, II – outer granular layer, III – outer pyramidal layer, IV – inner granular layer, V – inner pyramidal layer, and VI – polymorphic layer). Each cortical layer has a characteristic distribution of different neurons and their connections with other cortical and subcortical regions. There are direct connections between different cortical areas and indirect connections via the thalamus.
We have only relatively recently understood that a few regions of the brain can produce new neurons after birth, for instance, the olfactory bulb region, which controls our ability to smell; and the dentate region of the hippocampus, which plays a part in the formation of new memories. As well as subventricular zone (SVZ) of the lateral ventricle and the amygdala . This means that ‘the adult brain does grow neo-neurons after all!’ Pointing toward lifelong neuron formation in the human brain’s hippocampus, with implications of memory and disease.
Although the brain continues to display certain more subtle forms of plasticity in adulthood, ‘it is never as malleable as in the childhood.’
(2) Postnatal (After-Birth) Development
(i) At Birth
The evolutionarily old parts (reptilian brain) that regulate vital functions, such as respiration, cardiac rhythm, eating, sleeping, and eliminating metabolic waste products, are the most developed parts of the neonates. Interestingly, the evolutionarily new cerebral cortex (neo-cortex) that subserves higher-order functions, such cognition and behavior, is the least developed part of the brain.
(ii) First 18 Months of Life
(a) Synaptogenesis: Although most neurons are produced while we are still in the womb, the connections of neurons, known as synaptogenesis, occur postnatally. This happens at different rates in different parts of the brain. Synaptogenesis involves the growth of axons and dendrites that connect with each other creating new synapses. This process is mainly driven by the infant’s experiences; and occurs throughout the brain but notably in the previously underdeveloped cerebral cortex (Figure 4; Box -1) [5].
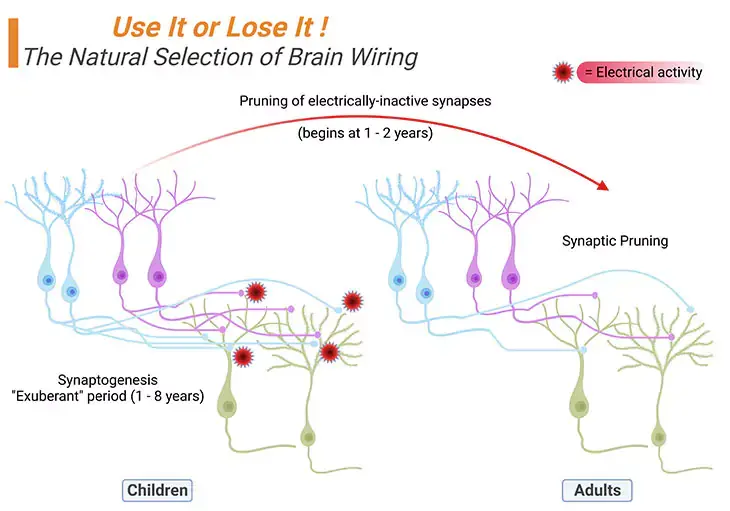
Figure 4. The Natural Selection of Brain Wiring: Synaptogenesis → Synaptic Pruning. During the ‘exuberant’ period of brain development, children generate about twice as many synapses (exhibited as small light pink/blue solid circles at the terminals of the axons) as they will eventually need, viz., synaptogenesis. Experience and/or electrical activity, will eventually determine which synapses will be preserved and which ones will be eliminated, viz., synaptic pruning. Thus, in general, no new neurons are added after birth, but new dendrites and synapses sprout furiously during a child’s earliest years, causing the cerebral cortex to thicken and its circuitry to grow massively more complex.
For example, on the one hand, the visual cortex gets wired together pretty soon and achieves its maximal synaptic density (i.e., the most connections it will ever have) around four months. On the other hand, the prefrontal cortex does not get to its maximal density until the child is about four years. Most of all, the other extraordinary thing that happens to the connections in the brain is that we produce far more of them than we need. As a result, by about the age of two years we have around 50% more connections in our brains that we typically have as an adult.
This means that, the proliferation of new nerve fibers (grey matter, body of neurons) is accompanied by increases in supportive and protective glial cells (white matter, myelinated axons), as a consequence of these combined growth processes the weight of the brain normally doubles between birth and 18 months.
(b) Synaptic Pruning: While there is a great deal of synaptogenesis during the first few years of life, thereafter, there is also a considerable amount of synaptic pruning. A process, in which the less-used axonal pathways are eliminated and solely the most-used pathways are retained (Figure 4; Box – 1).
Synaptic pruning has been likened to ‘moulding a sculpture from clay.’ We begin with excess clay, or neurons, and through a delicate process of refining and whittling down what is not needed, we are left with an object of beauty and efficiency. Synaptic pruning thus ensures the brain emerges into a smooth, well-oiled machine with connections that make sense.
The magnitude of this synaptic sorting is enormous. Children lose on the order of ‘20 billion synapses per day’ between early childhood and adolescence. While this may sound ruthless, it is generally a very beneficial thing. The elimination of stray synapses and the strengthening of survivors is what makes our mental processes more streamlined and coherent as we mature. Thus, it creates efficient channels for information transfer, and on the other hand it may also explain why our mental processes become less flexible and creative as we mature [6].
(c) Programmed Cell Death: Moreover, space for the proliferating axons and dendrites is made by the ‘programmed cell death or apoptosis,’ of some of the adjacent neurons. The prenatally formed surplus neuron ‘bank’ allows for this.
Ultimately, the combination of (a) synaptogenesis, accompanied by (b) synaptic pruning, and (c) programmed cell death is sometimes referred to as a process of ‘sculpting the brain’ into a functionally efficient form.
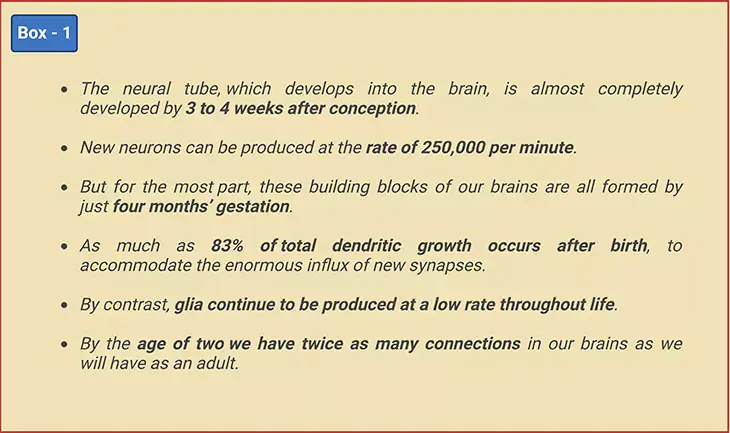
(d) Growth Spurts: Spurts in brain growth, with ensuing process of synaptic pruning and apoptosis, occur at approximately predictable ages from infancy through childhood through adolescence. The nature of these growth spurts is selective, building, and sculpting specific brain systems in turn (Figure 1, 3, & 4; Box – 1).
- The earliest spurt in brain growth occurs within in the first few months of life; when the cortical components of the visual and auditory systems are developed.
- One more growth spurt occurs at around age 2;0, surfaced by gains in language development.
- A further growth spurt occurs at around age 4:0, accompanied by a step change in the ability to ‘mindread,’ i.e., to think about one’s own thoughts and those of other individuals, an ability that is impaired in autism spectrum disorder (ASD).
- Further spurts of brain growth, predominantly involving increased connectivity between neurons in the frontal lobes and neurons in relative distant parts of the brain; persist through middle childhood to the late teenage years. And each accompanied by gradual improvements in increasingly sophisticated and human-specific abilities, for example, abstract reasoning and mathematical abilities.
- Additional growth may occur during adulthood if new skills or knowledge are acquired, thus providing evidence to support the phenomenon of ‘neural plasticity.’
From middle age onwards, though, there is a gradual loss of grey matter in specific brain regions, associated with a decline in cognitive abilities. This deterioration may be exacerbated by age-related disease such as dementia.
(e) Myelination: The process by which the axons of neurons are covered with an insulating layer designed to increase the neuron’s efficiency. Myelin is a fatty substance that grows around the axons of neurons as they develop. It is generated by oligodendrocytes. This insulates the body of the neurons and so speeds up transmission of signals along the axon. It develops in different regions starting with the brain stem at about 29 weeks. It tends to happen from inferior to superior, and posterior to anterior; meaning that it happens from bottom to top and from the back areas to the front. Myelination occurs mostly during childhood but in some area continues into adolescence and even beyond [7].
As the connections in the head develop (by implication brain size and volume), so the size of the head itself needs to grow! It expands nearly 14 cm on average during the first two years of life outside the womb; and followed by a further 7 cm during early childhood and adolescence.
Like many other areas of development, brain growth is not smooth and continuous; rather it occurs in spurts as mentioned above. The brain undergoes several growth spurts over the course of its development. Recording of brain electrical activity as reflected in electroencephalograms (EEG) appears to show correlations between growth spurts and major periods of cognitive development. For example:
- At 3 to 4 months – surges in frontal lobe activity that occur during infancy and toddlerhood appear to map onto the emergence of specific abilities; at 3 to 4 months when infants typically reach for objects;
- Around 8 months – when they typically crawl and search for hidden objects;
- At 12 months – when they typically walk; and
- Around 18 months – when infants language blossoms;
- Under 2 years – a surge in the production of synapses may account for these brain growth spurts in children.
While it may be tempting to draw meaningful connections between developments within the brain itself (i.e., changes due to synaptogenesis, synaptic pruning, myelination, and development of the frontal lobe) and cognitive development, however, there exists a caveat in doing so, arguing the fact that just studying global brain changes in brain structure is less informative than examining how regional developments in the brain develop to support specific functions.
(f) Lateralization: An important aspect of brain development that begins prenatally and continues from infancy is ‘laterization.’ As we know, the cerebrum and cerebellum are divided into left and right divisions. Subcortical structures are also bilaterally paired. The left and right components of a pair of structures usually subserve different but complementary functions.
For example, the auditory cortex that is located in the left cerebral hemisphere receives sound coming predominantly from the right ear, while the auditory cortex situated in the right hemisphere receives sound coming predominantly from left ear. Likewise, speech production is typically carried out in the left hemisphere, however prosody (the emotion bearing patterns of pitch and intonation) is generally carried out in the right hemisphere.
(3) Nature versus Nurture: What Drives Neurotypical Brain Development
Brain development is eventually governed by the activities of ‘genes (nature)’ and their products, including many of the neurochemicals discussed in our previous two blogs (cf. previous blogs entitled as ‘The Neurotypical Brain versus the Autistic Brain: Neurotypical Brain Chemistry’ and ‘The Neurotypical Brain versus the Autistic Brain: Brain Chemistry in ASD’). In any case, ‘environmental factors (nurture)’ are also equally important. For example, certain neurotoxins such as lead, to which a developing child may be exposed pre- or postnatally have adverse effects on brain development [8].
Most importantly, and on the positive side:
- Synaptogenesis is driven by (a) sensory, (b) motor, (c) social, and (d) emotional experiences that stimulate the growth of dendrites and axons.
- Increasing brain connectivity and building and reinforcing the brain circuits (regional and global) that will eventually subserve an increasing wide repertoire of abilities and behaviors.
In any case, the chemical processes involved in synaptogenesis are, however, mediated by gene expression products. Furthermore, the processes of pruning and programmed cells death (apoptosis) are also genetically controlled. Nevertheless, environmental factors also have an essential role in brain sculpting because experience determines which neuronal connections are well established (and consequently not pruned) as opposed to those that are poorly established (and consequently pruned).
This sort of interplay between genetic and environmental factors is typical of the process of brain development and change throughout the lifespan, ensuring that every neurotypical individual is unique.
Despite the above-mentioned facts, there is still some difference of opinion concerning the balance between the contributions of nature (as dictated by genes), and nurture (influenced by experience), particularly concerning the extent to which genes control precisely what circuits are built, and in which parts of the brain. This dialogue is absolutely relevant to theories of the immediate causes of the Socio-Emotional-Communicative (SEC) impairments and Restricted, Repetitive-Behaviors (RRBs) that are diagnostic of autism spectrum disorders (ASD); also to discussions of the extent to which ASD may or may not be ‘ameliorated’ or even for that matter ‘cured’ [9].
II. Neurotypical Brain Function
The whole brain functions as a hierarchy of nested systems referred to as neural circuits, systems, or networks. A network is a connection of many brain regions that interact with each other to give rise to a particular function. Every network consists of interconnected nuclei that operate together to subserve a particular function. Clusters of neurons, all of which are involved in transmitting and receiving the same information, are called nuclei (singular: nucleus).
Those neural circuits that involve relatively few nuclei and are situated close together in the brain are referred to as local networks. These networks carry out highly specific functions, for example, registering the color or shape of an object. However, if all salient features of an object simultaneously and as a whole or entirety, such as color, shape, size, and movement of an object or of a bird, in that scenario, activity in several local networks must be coordinated in a global network.
Certain global networks are very widely distributed within the brain, involving both left and right cerebral hemispheres. As an example, the hearing brain! Hearing is mediated by the auditory nerves that connect mechanisms in the left and right inner ears to nuclei in the brain stem; and these nuclei further connect to the primary hearing centers located in the left and right temporal lobes, and thereafter to adjacent auditory association areas in each temporal lobe.
To function efficiently:
- The various components of any network, whether local or global, must be connected with each other.
- The required connectivity is dependent on the availability and integrity of grey matter and white matter of the brain (the cell bodies of neurons are greyish-brown in color, and constitute grey matter, glial cells are whiteish in color, and constitute white matter in the brain).
- The neurochemicals that transmit and modulate ‘information’ within a network.
- Furthermore, the coherence of activity within any local or global network is dependent on the synchronization of the firing of groups of neurons within the network (neurons that wire together, fire together).
- Eventually, the synchronized activity of large groups of neurons gives rise to rhythmic ‘neural oscillations’ or ‘brain waves’ of various frequencies.
The neural oscillations are named with letters of the Greek alphabet, such as alpha, beta, gamma, etcetera; as discussed below and corresponding brain waves are schematically illustrated in Figure 5 [10].
Alpha waves are neural oscillations in the brain with a frequency range of 7.5 – 12 Hz. This rhythm probably originating from the synchronous and coherent electrical activity of thalamic pacemaker cells in humans. Alpha waves are detected at different stages of the wake-sleep cycle. The alpha activity is centered in the occipital lobe, although it has been speculated that it has a thalamic origin.
Beta waves are neural oscillations in the brain with a frequency range of 12 – 30 Hz. Beta rhythm is associated with normal waking consciousness. Beta waves often conceived indicative of inhibitory cortical transmission medicated by gamma aminobutyric acid (GABA), which is the predominant inhibitory neurotransmitter of the mammalian nervous system. Consequently, beta waves are possibly biomarkers of GABAergic dysfunction, especially in neurodevelopmental disorders caused by genetic defects such as 15q deletions or duplications.
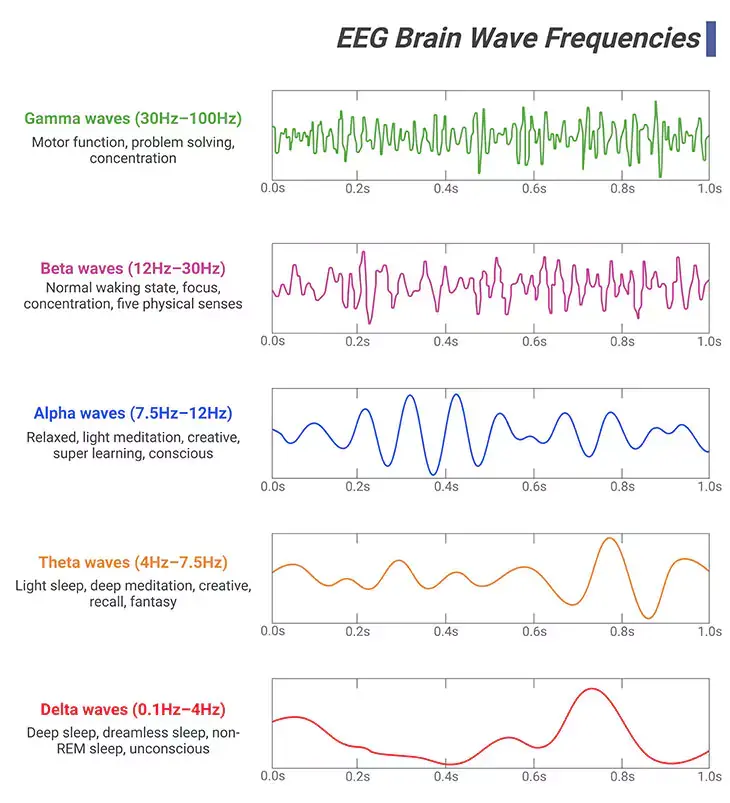
Figure 5. Neural Oscillations. ‘Brain or neural oscillations’ refers to the rhythmic and/or repetitive electrical activity generated spontaneously and in response to stimuli by neural tissue in the central nervous system. It can be recorded using various electrophysiological methods, such as electroencephalogram (EEG) or magnetoencephalogram (MEG); recorded either invasively or non-invasively, i.e., from inside the brain or from electrodes attached to the scalp. There are at least ten discrete brain rhythms, covering more than four orders of magnitude in frequency, from approximately 0.02 to 600 hertz (Hz). Most of the oscillations are short-lived, i.e., less than a second, while others can be sustained for longer periods. In humans: (i) Gamma wave is a pattern of neural oscillations with a frequency between 30 to 100 Hz; associated with motor function, problem solving, and concentration. (ii) Beta wave is a pattern of neural oscillations with a frequency between 12 to 30 Hz; linked to normal waking state, focus, concentration, and five physical senses. (iii) Alpha wave is a pattern of neural oscillations with a frequency between 7.5 to 12 Hz; correlated with relaxed, light meditation, creative, super learning, and consciousness. (iv) Theta wave is a pattern of neural oscillations with a frequency between 4 to 7.5 Hz; associated with light sleep, deep meditation, creative, recall, and fantasy. (v) Delta wave is a pattern of neural oscillations with a frequency between 0.1 to 4 Hz; associated with deep sleep, dreamless sleep, non-REM sleep, and unconscious.
Gamma waves are neural oscillations in the brain with a frequency range of 30 – 100 Hz. Gamma rhythms are correlated with large-scale brain network activity, including cognitive phenomena, for example, working memory, attention, and perceptual groupings. Altered gamma activity has been linked to certain mood and cognitive disorders, such as major depressive or bipolar disorders, schizophrenia, epilepsy, and Alzheimer’s disease. In addition, hypersensitivity and memory deficits due to fragile X syndrome (FXS) may be associated to gamma rhythm anomalies in the sensory cortex and hippocampus.
Delta waves are high amplitude neural oscillations in the brain with a frequency range of 0.1 – 4 Hz. Delta rhythms are typically associated with the deep stage 3 of non-REM sleep (slow-wave sleep, SWS). And it assists in characterizing the depth of sleep. As a result, suppression of delta waves drives to inability of body rejuvenation, brain revitalization, and poor sleep.
Theta waves are neural oscillations in the brain with a frequency range of 4 – 7.5 Hz. Theta rhythms underpin various aspects of cognition and behavior, including learning, memory, and spatial navigations in many animals. Broadly, two types of theta rhythm have been described.
- The ‘hippocampal theta rhythm (96 – 10 Hz)’ is a strong oscillation that can be observed in the hippocampus and other brain regions connected to it, in numerous mammalian species.
- While ‘cortical theta rhythm (4 – 7 Hz)’ is low-frequency components of scalp EEG, usually recorded from human beings, regardless of where in the brain they occur or what their functional significance is.
Summary and Conclusions
In this blog, we have explored in a succinct manner the neurotypical brain development and function. It can be argued that all behavior, thoughts, and feelings are rooted in neural events, and consequently that understanding how the brain develops holds the key to unlocking the essential mysteries of human development, including why and how we grow up to learn and behave the way we do. Moving beyond a description of neuroanatomy, we have briefly examined the emerging field of developmental neuroscience, which is concerned with how the brain changes across development related to cognitive and often social and emotional functioning.
The take-home messages are: (a) almost all neurons are produced during the embryonic stage of development; (b) both genetic encoding and environmental factors determine development; (c) postnatal synaptogenesis, neural plasticity, and the pruning of little-used or unused connections all make the brain a highly adaptable and experience-dependent organ; and (d) the brain undergoes a significant set of changes during adolescence.
For information on autism monitoring, screening and testing please read our blog.
References
- Copp AJ, Greene ND, Murdoch JN. The genetic basis of mammalian neurulation. Nat Rev Genet. 2003 Oct;4(10):784-93. doi: 10.1038/nrg1181. PMID: 13679871.
https://pubmed.ncbi.nlm.nih.gov/13679871/ - Clouchoux C, Guizard N, Evans AC, du Plessis AJ, Limperopoulos C. Normative fetal brain growth by quantitative in vivo magnetic resonance imaging. Am J Obstet Gynecol. 2012 Feb;206(2):173.e1-8. doi: 10.1016/j.ajog.2011.10.002. Epub 2011 Oct 12. PMID: 22055336.
https://pubmed.ncbi.nlm.nih.gov/22055336/ - Merkle FT, Alvarez-Buylla A. Neural stem cells in mammalian development. Curr Opin Cell Biol. 2006 Dec;18(6):704-9. doi: 10.1016/j.ceb.2006.09.008. Epub 2006 Oct 12. PMID: 17046226.
https://pubmed.ncbi.nlm.nih.gov/17046226/ - Götz M, Huttner WB. The cell biology of neurogenesis. Nat Rev Mol Cell Biol. 2005 Oct;6(10):777-88. doi: 10.1038/nrm1739. PMID: 16314867.
https://pubmed.ncbi.nlm.nih.gov/16314867/ - Huttenlocher PR. Synaptic density in human frontal cortex – developmental changes and effects of aging. Brain Res. 1979 Mar 16;163(2):195-205. doi: 10.1016/0006-8993(79)90349-4. PMID: 427544.
https://pubmed.ncbi.nlm.nih.gov/427544/ - Eltokhi A, Janmaat IE, Genedi M, Haarman BCM, Sommer IEC. Dysregulation of synaptic pruning as a possible link between intestinal microbiota dysbiosis and neuropsychiatric disorders. J Neurosci Res. 2020 Jul;98(7):1335-1369. doi: 10.1002/jnr.24616. Epub 2020 Apr 2. PMID: 32239720.
https://pubmed.ncbi.nlm.nih.gov/32239720/ - Dubois J, Dehaene-Lambertz G, Kulikova S, Poupon C, Hüppi PS, Hertz-Pannier L. The early development of brain white matter: a review of imaging studies in fetuses, newborns and infants. Neuroscience. 2014 Sep 12;276:48-71. doi: 10.1016/j.neuroscience.2013.12.044. Epub 2013 Dec 28. PMID: 24378955.
https://pubmed.ncbi.nlm.nih.gov/24378955/ - Stiles J. Brain development and the nature versus nurture debate. Prog Brain Res. 2011;189:3-22. doi: 10.1016/B978-0-444-53884-0.00015-4. PMID: 21489380.
https://pubmed.ncbi.nlm.nih.gov/21489380/ - Johnson MH, Jones EJ, Gliga T. Brain adaptation and alternative developmental trajectories. Dev Psychopathol. 2015 May;27(2):425-42. doi: 10.1017/S0954579415000073. PMID: 25997763.
https://pubmed.ncbi.nlm.nih.gov/25997763/ - Doelling KB, Assaneo MF. Neural oscillations are a start toward understanding brain activity rather than the end. PLoS Biol. 2021 May 4;19(5):e3001234. doi: 10.1371/journal.pbio.3001234. PMID: 33945528; PMCID: PMC8121326.
https://pubmed.ncbi.nlm.nih.gov/33945528/