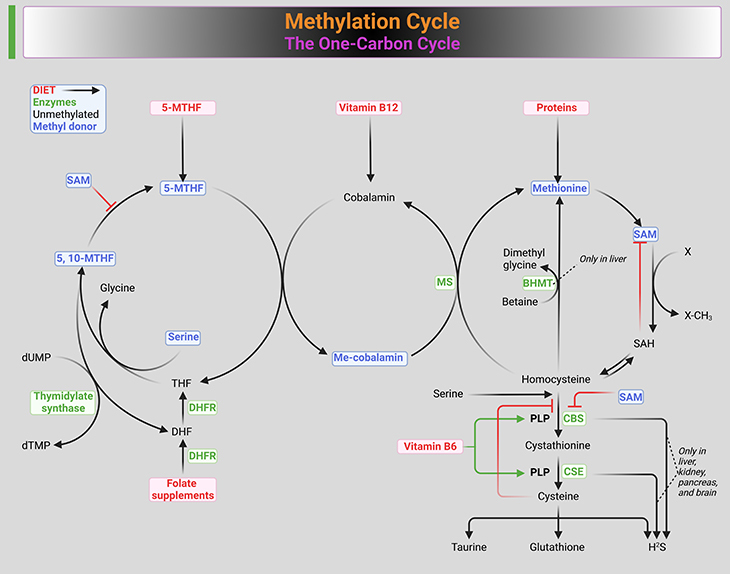
Figure 1. Methylation cycle. Illustration of the ‘one-carbon metabolism pathways,’ encompassing the folate, methionine, and transulfuration cycles. (1) Folate Pathway: The folate pathway begins with dietary folates being converted to dihydrofolate (DHF) and then to tetrahydrofolate (THF). THF is further processed into 5,10-methylene-THF, which provides a methyl group to convert homocysteine to methionine, with the help of the enzyme methionine synthase and vitamin B12. The reaction also produces 5-methyl-THF. This cycle is crucial for DNA synthesis and repair. (2) Methionine Pathway: Dietary methionine combines with adenosine triphosphate (ATP) to form S-adenosylmethionine (SAMe), a key methyl donor for biological methylation reactions. After donating its methyl group, SAMe is converted into S-adenosylhomocysteine (SAH) and then into homocysteine. This pathway is essential for generating methyl groups required for methylation reactions, including DNA and protein methylation. (3) Transulfuration Pathway: Homocysteine is subsequently either recycled back to methionine or converted into cystathionine via the enzyme cystathionine beta-synthase (CBS). Cystathionine is further broken down into cysteine, which is a precursor for glutathione, an important antioxidant. This pathway is critical for maintaining cellular redox balance and detoxification processes. (4) Key Enzymes and Co-Factors: The figure highlights important enzymes such as methionine synthase (MS), cystathionine beta-synthase (CBS), and dihydrofolate reductase (DHFR), and essential co-factors like vitamin B12 and folate (B9) derivatives. It depicts the interconnected nature of these pathways in maintaining proper methylation balance and overall metabolic health. This detailed figure legend outlines the intricate biochemistry of one-carbon metabolism, emphasizing its significance in supporting vital cellular processes through folate, methionine, and transulfuration pathways.
Introduction
Methylation, a fundamental biochemical process, is pivotal to numerous bodily functions, impacting both physical and mental health. In essence, methylation involves the addition of a methyl group (CH3) to an atom or molecule, participating in countless chemical reactions within the body and brain. This process is critical for various aspects of human health, influencing personality traits, behavioral tendencies, and susceptibility to certain disorders [1].
Undermethylation is often linked with traits such as perfectionism, strong will, high achievement, obsessive-compulsive tendencies, and seasonal allergies. Conversely, overmethylation correlates with excellent social skills, numerous friendships, non-competitiveness, artistic interests, chemical sensitivities, and a tendency toward anxiety. Methylation imbalances can contribute to numerous serious conditions, affecting millions globally.
The emerging field of epigenetics is revolutionizing our understanding of conditions like cancer, heart disease, mental illness, and autism. It is now evident that many disorders previously deemed genetic and untreatable are actually epigenetic and highly manageable. This realization underscores the importance of understanding methylation, as it is the dominant chemical factor in epigenetics. Consequently, approaches to normalize methylation are poised to become a primary focus of medical research over the coming decades [2-3].
This article delves into the prevalence of methylation disorders, the various methylation reactions vital for life, and the importance of methylation lab testing. By exploring the one-carbon cycle, the cornerstone of methylation (see Figure 1), we can comprehend how this biochemical process influences health and behavior [4].
Understanding methylation not only offers insights into gene regulation but also highlights the profound impact of epigenetic mechanisms on our lives. As we uncover the intricacies of this process, we pave the way for novel therapeutic interventions and a deeper appreciation of how our environment shapes our biology. (Cf. previous blogs entitled as: “Developmental Origins of Health and Disease: Epigenetics, Nutrition, and Infant Health.” “Generational Epigenetics: How Nutrition and Environment Shape Lifelong Brain Development.”)
Prevalence of Methylation Disorders
Drawing from a database of 30,000 individuals, it has been observed that about 70% exhibit normal methylation, 22% are undermethylated, and 8% are overmethylated. However, more than two-thirds of individuals diagnosed with behavioral or mental disorders exhibit a methylation imbalance. Methylation status is established during the first few months of in-utero development and typically persists throughout life. There are four primary types of methylation reactions essential to human life:
- Methylation of Atoms: An important reaction in humans is the methylation of metals, such as mercury and other toxic metals, which impacts their availability to the brain and other organs and influences the rate of excretion from the body.
- Methylation of Molecules: Hundreds of biochemical reactions involve the donation of a methyl group to a molecule, often facilitated by a methyltransferase enzyme. For instance, methylation of amino acids is crucial for producing various proteins in the body (see Figure 1).
- DNA Methylation: Gene expression within cells is regulated by methylation at specific cytosine sites along the DNA double-helix molecule. This reaction primarily occurs at CpG sites, where a guanine residue is adjacent to cytosine, connected by a phosphorous atom. CpG methylation generally inhibits gene expression, affecting the rate of protein synthesis in individual cells and tissues.
- Methylation of Histone Proteins: Gene expression is also regulated through the methylation or acetylation of histones, the proteins that support fragile DNA strands in the cell nucleus. Histone methylation usually compacts the DNA, restricting the access of transcription factors necessary for gene expression. However, methylation at certain histone sites can promote gene expression (see Figure 2).
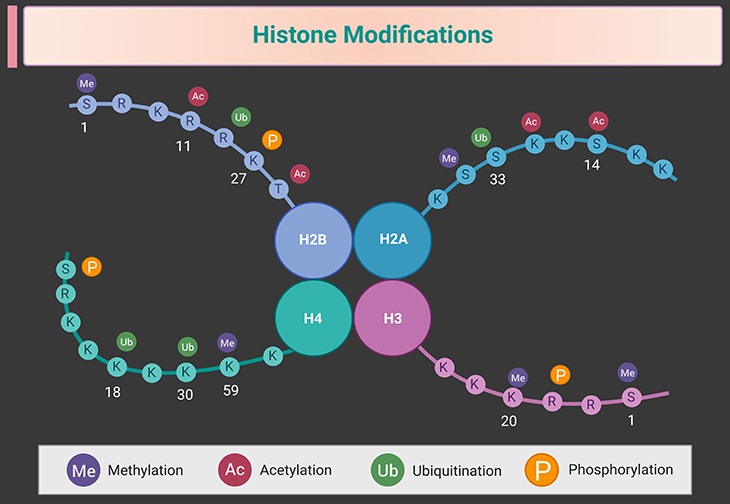
Figure 2. Histone modifications. Illustration depicting histone modifications, including methylation, acetylation, phosphorylation, ubiquitination, and sumoylation. (1) Histone Methylation: The addition of methyl groups (CH3) to histone proteins, primarily at lysine (K) and arginine (R) residues, by histone methyltransferases (HMTs). Methylation can either activate or repress gene expression depending on the specific amino acid residue modified and the number of methyl groups added (mono-, di-, or tri-methylation). For example, tri-methylation of lysine 27 on histone H3 (H3K27me3) is associated with gene silencing. (2) Histone Acetylation: The addition of acetyl groups (CH3CO) to lysine (K) residues on histone tails by histone acetyltransferases (HATs). This modification neutralizes the positive charge on histones, reducing their affinity for negatively charged DNA, and thereby promoting a more relaxed chromatin structure that is accessible for transcription. Deacetylation by histone deacetylases (HDACs) reverses this process and often leads to transcriptional repression. (3) Histone Phosphorylation: The addition of phosphate groups (PO4) to serine (S), threonine (T), or tyrosine (Y) residues by kinases. Phosphorylation is associated with chromatin condensation and relaxation, and plays critical roles during cell division, DNA damage response, and transcriptional regulation. (4) Histone Ubiquitination: The attachment of ubiquitin (a small regulatory protein) to lysine (K) residues by E3 ubiquitin ligases. Ubiquitination can signal for histone degradation, affect nucleosome dynamics, and modulate access to DNA, thereby playing roles in gene expression regulation and DNA repair. (5) Histone Sumoylation: The addition of small ubiquitin-like modifier (SUMO) proteins to histones, typically linked with transcriptional repression. Sumoylation often acts antagonistically to acetylation and can influence chromatin structure and protein interactions. (6) Key Enzymes and Impact: The figure outlines the key enzymes involved in each modification, such as HMTs, HATs, HDACs, kinases, and ubiquitin ligases. It also displays the dynamic nature of histone modifications and how they influence the structure of chromatin and regulate gene expression, impacting various biological processes. This figure legend provides a comprehensive overview of the crucial histone modifications and their roles in regulating gene activity and maintaining cellular function.
Methylation Lab Testing
A person’s methylation status is influenced by diet and environment, but genetics is usually the dominant factor. Most methyl groups originate from dietary methionine, which converts to S-adenosylmethionine (SAMe), an unstable molecule that donates methyl groups for essential reactions. The production and regulation of SAMe are managed by a complex ‘one-carbon cycle’ or ‘methylation cycle’ of biochemical reactions, dependent on numerous enzymes prone to genetic mutations.
For example, the important MTHFR enzyme, which contains more than 500 amino acids and has a molecular weight exceeding 77,000, is susceptible to mutations [5]. Single nucleotide polymorphisms (SNPs) can alter the enzyme’s function, markedly reducing SAMe production and resulting in undermethylation. Overmethylation can occur from inefficient SAMe utilization, especially in the creatine pathway. Typically, over 70% of SAMe produced in the one-carbon cycle is consumed in creatine production. SNP mutations in this process can lead to excess unused SAMe throughout the body.
Since some SNPs reduce methylation while others increase it, a patient’s overall methylation status depends on the combined impact of these SNPs. Popular genetic tests for MTHFR, MS, and other SNPs are qualitative and limited in determining overall methylation status. Two lab assays, the SAMe/SAH ratio and whole-blood histamine, directly measure the net effect of competing SNPs.
The One-Carbon Cycle
A versatile methyl donor, SAMe, is found in every cell in the human body. SAMe is produced from methionine, an amino acid present in dietary protein. A stable SAMe concentration is essential for normal embryonic development and chemical processes throughout life. The one-carbon cycle, also known as the SAM cycle or methylation cycle, regulates SAMe levels through a series of chemical reactions that produce, consume, and regenerate SAMe.
- Step 1: Dietary methionine combines with adenosine triphosphate (ATP) to form SAMe, assisted by magnesium.
- Step 2: After donating its methyl group, SAMe transforms into S-adenosylhomocysteine (SAH). Efficient removal of SAH by chemical reactions ensures proper methylation.
- Step 3: In healthy individuals, SAH converts to homocysteine (Hcy), maintaining proper methylation levels. This reaction involves an enzyme that removes the adenosine group.
- Step 4: Homocysteine is recycled to methionine or converted to cystathionine, both essential for good health. High oxidative stress can cause undermethylation and vice versa. Recycled Hcy to methionine reactions are enabled by vitamins such as 5-methyltetrahydrofolate (5-MeTHF) and B12.
Understanding methylation and the one-carbon cycle is integral to advancing medical research and treatment, particularly in the dynamic field of epigenetics [6]. (Cf. previous blogs entitled as: “Developmental Delays in Infancy and Early Childhood: Metabolism of Homocysteine.” “Folate in Health and Disease: Genetic Defects in Folate Pathway Influencing the Central Nervous System.”)
Take-Home Messages
Methylation
- Vital biochemical process involving the addition of a methyl group (CH3) to an atom or molecule, essential for numerous bodily and brain functions.
Physical and Mental Health
- Methylation status influences traits and behaviors, with undermethylation linked to perfectionism and OCD tendencies, while overmethylation is associated with social skills and anxiety.
Epigenetics
- Plays a critical role in understanding conditions like cancer and mental illness, with many disorders previously thought to be genetic now deemed epigenetic and treatable.
Methylation Types
- Includes methylation of atoms, molecules, DNA (CpG sites), and histone proteins, regulating gene expression and impacting health.
Lab Testing
- Genetic factors dominate methylation status, which can be assessed through SAMe/SAH ratio and whole-blood histamine assays.
One-Carbon Cycle
- Essential biochemical pathway regulating SAMe levels, involving methionine from diet, with steps to ensure proper methylation and antioxidant production.
These distilled facts encapsulate the significance of methylation and its impact on health and behavior.
Summary and Conclusions
Methylation is a critical biochemical process that involves the addition of a methyl group (CH3) to atoms or molecules, playing a central role in various bodily functions including gene regulation and protein synthesis. This process significantly impacts physical and mental health by influencing traits and behaviors. For example, undermethylation is associated with high achievement and OCD tendencies, while overmethylation correlates with strong social skills and anxiety.
The emerging field of epigenetics, which studies how gene expression is regulated without changing the DNA sequence, is crucial for understanding conditions like cancer, heart disease, mental illness, and autism. As we gain further insight into methylation, future research is expected to focus on normalizing methylation imbalances, offering new therapeutic interventions for numerous conditions.
Methylation’s role in gene expression regulation through DNA and histone protein methylation emphasizes its importance in development and disease. Notably, methylation imbalances have been implicated in various mental disorders, including neurodevelopmental and neuropsychiatric conditions such as autism, ADHD, depression, and schizophrenia. These conditions often involve disrupted methylation patterns, which affect brain function and development, leading to symptoms that impact behavior, cognition, and emotional regulation.
The future of methylation studies and epigenetics looks promising, with advances in technology and scientific understanding paving the way for personalized medical treatments. These developments will enhance our ability to prevent, diagnose, and treat a variety of conditions, thereby improving health outcomes and expanding our knowledge of the complex interactions between our genes and the environment.
For information on autism monitoring, screening and testing please read our blog.
References
- Moore LD, Le T, Fan G. DNA methylation and its basic function. Neuropsychopharmacology. 2013 Jan;38(1):23-38. doi: 10.1038/npp.2012.112. Epub 2012 Jul 11. PMID: 22781841; PMCID: PMC3521964.
https://pubmed.ncbi.nlm.nih.gov/22781841/ - Korolenko A, Skinner MK. Generational stability of epigenetic transgenerational inheritance facilitates adaptation and evolution. Epigenetics. 2024 Dec;19(1):2380929. doi: 10.1080/15592294.2024.2380929. Epub 2024 Aug 5. PMID: 39104183; PMCID: PMC11305060.
https://pubmed.ncbi.nlm.nih.gov/39104183/ - Non AL. Social epigenomics: are we at an impasse? Epigenomics. 2021 Nov;13(21):1747-1759. doi: 10.2217/epi-2020-0136. Epub 2021 Mar 22. PMID: 33749316; PMCID: PMC8819598.
https://pubmed.ncbi.nlm.nih.gov/33749316/
- Froese DS, Fowler B, Baumgartner MR. Vitamin B12 , folate, and the methionine remethylation cycle-biochemistry, pathways, and regulation. J Inherit Metab Dis. 2019 Jul;42(4):673-685. doi: 10.1002/jimd.12009. Epub 2019 Jan 28. PMID: 30693532.
https://pubmed.ncbi.nlm.nih.gov/30693532/ - Long S, Goldblatt J. MTHFR genetic testing: Controversy and clinical implications. Aust Fam Physician. 2016 Apr;45(4):237-40. PMID: 27052143.
https://pubmed.ncbi.nlm.nih.gov/27052143/ - Villicaña S, Bell JT. Genetic impacts on DNA methylation: research findings and future perspectives. Genome Biol. 2021 Apr 30;22(1):127. doi: 10.1186/s13059-021-02347-6. PMID: 33931130; PMCID: PMC8086086.
https://pubmed.ncbi.nlm.nih.gov/33931130/