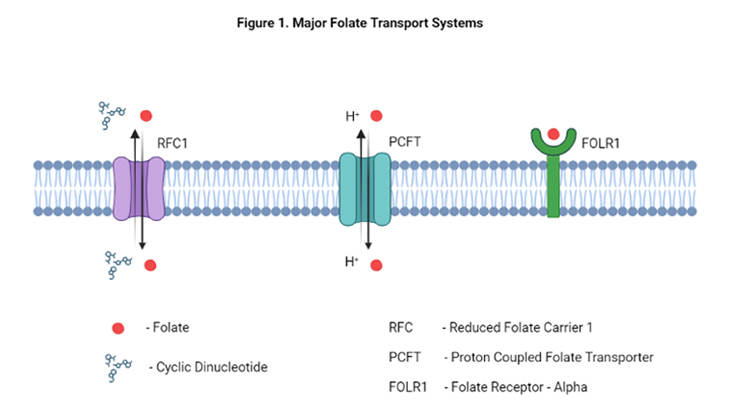
Introduction
Folate transport by mammalian tissues and cells has been under intense scrutiny and the subject of several research studies [1-2]. Here we present an overview of our current knowledge pertaining to folate transport systems; and aim to address the most fundamental question pertaining to folate absorption and transport from the intestinal tract to other parts of the body to start with, i.e., how do mammalian tissues can harvest folate (vitamin B9) from diet and circulating blood?
Mammalian tissues, including human tissues, harvest folate from the diet and circulating blood via several different folate transport systems. The primary mechanisms for folate delivery into the cell are through the following three processes, viz., carrier-mediated, receptor-mediated, and transport-mediated.
- Carrier-mediated involves the reduced folate carrier 1 (RFC1)
- Transport-mediated occurs through the proton-coupled folate transporter (PCFT)
- Receptor-mediated happens through folate receptors α, β, and γ (FOLR1, FOLR2, and FOLR3)
All these three transport systems are distinguished by their distinctive patterns of tissue expression, divergent specificities for reduced versus oxidized folates, as well as differing protein-structures and mechanisms for the transmembrane transport of folates.
Thus far, a number of different folate transport systems have been described that falls into two major classes (Table 1).
Folate Transport Systems | Gene Symbol | |
---|---|---|
A. Transmembrane carriers | ||
1. | Reduced folate carrier 1 (RFC1) | SLC19A1 |
2. | Proton coupled folate transporter (PCFT) | SLC46A1 |
3. | Mitochondrial folate transporter (MFT) | SLC25A32 |
4. | Lysosomal folate transporter (LFT) | – |
5. | ATP binding cassette exporters (ABC/MRPs) | MRP1-MRP5 |
6. | Other exporters | SLC21, SLC22 |
B. Folate receptors | ||
1. | Folate receptor-α, adult | FOLR1 |
2. | Folate receptor-β, fetal | FOLR2 |
3. | Folate receptor-γ | FOLR3 |
Table 1. Major classes of folate transport systems in human cells and tissues.
Here, we discuss the various characteristics of the first category of folate transport systems, viz., the transmembrane carriers; and then in our subsequent blog we will provide an overview about current developments pertaining to various types of folate receptors, the second category.
Transmembrane carriers
The etiology of ASD is complex and recent research has shed light on the role of genetic factors in the development of ASD.
One of the most significant findings in ASD research is the high heritability of the disorder. Twin studies have consistently shown that ASD has a strong genetic component, with heritability estimates ranging from 64% to 91% (Hallmayer et al., 2011, Tick et al., 2016). Several large-scale genome-wide association studies (GWAS) have identified multiple common genetic variants associated with ASD, including variants in genes involved in neuronal development, synapse formation, and immune system function (Gaugler et al, 2014, Grove et al 2019)
Other research has also focused on rare genetic variants, such as copy number variations (CNVs) and de novo mutations, which are present in a small percentage of individuals with ASD but have a large effect on ASD risk. Several studies have identified specific CNVs and de novo mutations that are strongly associated with ASD, including deletions or duplications in the 16p11.2 and 22q11.2 regions and mutations in the CHD8 and SCN2A genes (Sander et al., 2015).
A variety of transmembrane folate transporters have been characterized kinetically and thermodynamically not only in various types of tissues but also in several laboratory grown mammalian cells, in vitro. In addition, recently, their corresponding genes have been isolated and characterized, and their tissue-specific expression patterns have been explored meticulously.
1. Reduced Folate Carrier 1 (RFC1):
The major route of folate transport into mammalian cells is via RFC1. The SLC19A1 gene encodes RFC1, a bidirectional transmembrane anionic exchanger, characterized by 12 transmembrane domains. RFC1 functions optimally at physiological pH of 7.4 and essentially inactive below pH of 6.5. RFC1 has been the most extensively studied of all the folate transporters.
RFC1 is expressed in multiple types of tissues and preferentially transports reduced forms of folates. It is fairly ubiquitous and expressed in multiple normal adult tissues as well in various tissue culture cells. Besides, RFC1 is detected in some fetal tissues and tumor cells. Although RFC1 is ubiquitously expressed in normal adult tissue, it may not be responsible for the bulk of folate transport in all types of tissues. It is highly expressed in the following tissues, for example:
- The brush border of the small intestine and large intestine
- Basolateral membrane of hepatocytes, renal tubular epithelium, choroid plexus, and retinal pigment epithelium
- Placenta, yolk sac, neural tube, craniofacial region, limb buds, and heart
RFC1 is a facilitative carrier, and folate transport into cells is stimulated by high levels of transcellular organic anions such as adenine nucleotides, whereas extracellular organic anions are inhibitory [2-4]. Thus, the relatively high concentration of intracellular anions makes folate transport essentially unidirectional. The other two members of RFC1 gene family, viz., SLC19A2 and SLC19A3, are facilitative thiamin transporters and they do not transport folate. Although thiamin is not transported by RFC1, thiamine pyrophosphate is, besides, high intracellular concentrations of thiamin pyrophosphate stimulate folate transport into cells.
The RCF1 is saturable and has a fairly low affinity for folate with Kt values in the low micromolar range {Kt ≈ 3 μM} for reduced folates and a similar affinity for the antifolate agent methotrexate [3, 5]. This carrier has a significantly reduced affinity for folic acid {Kt ≈ 200 μM}, and this clearly explains why most in vitro cultured cells require much higher levels of folic acid compared with reduced folates for cellular growth.
2. Proton Coupled Folate Transporter (PCFT):
The proton coupled folate transporter (PCFT) is a recently identified bidirectional transmembrane folate carrier and is encoded by the SLC46A1 gene in humans. There are two other members in this family (SLC46A2 and SLC46A3), but their functions are not known.
PCFT functions optimally at acidic pH and reduced or negligible activity at pH of 4.5, depending on the folate form [6-7]. At acidic pH, proton coupling enables the concentrative transport of folates. Therefore, the major physiological roles of PCFTs are in mediating the intestinal absorption of folate and reduced folates, and its delivery to the central nervous system. PCFT gene is highly expressed in the following tissues, for instance:
- Gastrointestinal tract, especially in the jejunum and duodenum of the small intestine (selectively, brush-border membrane and apical cytoplasm of duodenum)
- Liver, spleen, kidney, prostate, breast, brain, placenta, retina, and retinal pigment epithelium, as well as expressed in other tissues
Unlike RFC1, PCFT carrier has essentially similar affinity for both reduced folates and folic acid {Kt = 0.5 to 0.8 μM at pH 5.5}. In addition, 5-methyl-THF is transported more effectively than folic acid at a pH of 6.5.
3. Mitochondrial Folate Transporter (MFT):
The mitochondrial folate transporter (MFT) is a transmembrane transporter protein that facilitates the uptake of folate by the mitochondrion; and is distinct from the other folate transporters. It is encoded by the SLC25A32 gene and belongs to the mitochondrial carrier superfamily [9]. MFT is specific for reduced folates; however, folic acid and methotrexate (an antimetabolite/antifolate agent) are not transported.
4. Lysosomal Folate Transporter (LFT):
A transporter that is responsible for methotrexate polyglutamate uptake by lysosomes has been reported but received little further attention [10]. However, the role, if any, of this transporter in folate transport or turnover is not known, at present.
5. ATP Binding Cassette Exporters (ABC):
A family of low-affinity membrane carriers, including multidrug resistance-associated protein (MRP1-MRP5) (ABCC1-ABCC5) [2, 8], can serve as folate exporters from tissues. These proteins were originally identified being responsible for multidrug resistance of cells to various antibiotics and anticancer agents by pumping drugs out of the cells. A common mechanism is the overexpression of ABC exporters, such as P-glycoprotein (P-gp/ABCB1), multidrug resistance-associated protein 2 (MRP2), and breast cancer resistance protein (BCRP/ABCG2), in cancer cells that limit the exposure to chemotherapeutic agents. The affinity of folates for these carriers is very low {Kt = 0.2 – 2mM}, but they have high capacity for folate transport.
6. Other Exporters:
Apart from this, there are several other organic anion transporters (OAT) that belong to solute carrier (SLC) group of membrane transport proteins. Most of the members of the SLC group are located in the cell membrane. Several SLC families are members of the major facilitator superfamily. Thus, a number of these OATs, such as the solute carriers SLC21 and SLC22, may potentially play a role in folate transport or efflux [2].
Conclusion
In this report, we have summarized our current understanding of the properties of various folate transporters that are responsible for importing folate (influx) into the tissue or exporting folate (efflux) from the tissues under physiological conditions. However, our understanding of potential defects and/or dysfunctions in these transport mechanistic processes under pathological conditions is more limited at present, and largely unknown.
For information on autism monitoring, screening and testing please read our blog.
References
- Sirotnak FM, Tolner B. Carrier-mediated membrane transport of folates in mammalian cells. Annu Rev Nutr. 1999;19:91-122. doi: 10.1146/annurev.nutr.19.1.91. PMID: 10448518.
https://pubmed.ncbi.nlm.nih.gov/10448518/ - Zhao R, Matherly LH, Goldman ID. Membrane transporters and folate homeostasis: intestinal absorption and transport into systemic compartments and tissues. Expert Rev Mol Med. 2009 Jan 28;11:e4. doi: 10.1017/S1462399409000969. PMID: 19173758; PMCID: PMC3770294.
https://pubmed.ncbi.nlm.nih.gov/19173758/ - Visentin M, Diop-Bove N, Zhao R, Goldman ID. The intestinal absorption of folates. Annu Rev Physiol. 2014;76:251-74. doi: 10.1146/annurev-physiol-020911-153251. PMID: 24512081; PMCID: PMC3982215.
https://pubmed.ncbi.nlm.nih.gov/24512081/ - Matherly LH, Hou Z, Deng Y. Human reduced folate carrier: translation of basic biology to cancer etiology and therapy. Cancer Metastasis Rev. 2007 Mar;26(1):111-28. doi: 10.1007/s10555-007-9046-2. PMID: 17334909.
https://pubmed.ncbi.nlm.nih.gov/17334909/ - Zhao R, Gao F, Goldman ID. Reduced folate carrier transports thiamine monophosphate: an alternative route for thiamine delivery into mammalian cells. Am J Physiol Cell Physiol. 2002 Jun;282(6):C1512-7. doi: 10.1152/ajpcell.00547.2001. PMID: 11997266.
https://pubmed.ncbi.nlm.nih.gov/11997266/ - Hou Z, Gangjee A, Matherly LH. The evolving biology of the proton-coupled folate transporter: New insights into regulation, structure, and mechanism. FASEB J. 2022 Feb;36(2):e22164. doi: 10.1096/fj.202101704R. PMID: 35061292; PMCID: PMC8978580.
https://pubmed.ncbi.nlm.nih.gov/35061292/ - Qiu A, Jansen M, Sakaris A, Min SH, Chattopadhyay S, Tsai E, Sandoval C, Zhao R, Akabas MH, Goldman ID. Identification of an intestinal folate transporter and the molecular basis for hereditary folate malabsorption. Cell. 2006 Dec 1;127(5):917-28. doi: 10.1016/j.cell.2006.09.041. PMID: 17129779.
https://pubmed.ncbi.nlm.nih.gov/17129779/ - Kruh GD, Belinsky MG. The MRP family of drug efflux pumps. Oncogene. 2003 Oct 20;22(47):7537-52. doi: 10.1038/sj.onc.1206953. PMID: 14576857.
https://pubmed.ncbi.nlm.nih.gov/14576857/ - Titus SA, Moran RG. Retrovirally mediated complementation of the glyB phenotype. Cloning of a human gene encoding the carrier for entry of folates into mitochondria. J Biol Chem. 2000 Nov 24;275(47):36811-7. doi: 10.1074/jbc.M005163200. PMID: 10978331.
https://pubmed.ncbi.nlm.nih.gov/10978331/ - Barrueco JR, O’Leary DF, Sirotnak FM. Metabolic turnover of methotrexate polyglutamates in lysosomes derived from S180 cells. Definition of a two-step process limited by mediated lysosomal permeation of polyglutamates and activating reduced sulfhydryl compounds. J Biol Chem. 1992 Aug 5;267(22):15356-61. PMID: 1379226.
https://pubmed.ncbi.nlm.nih.gov/1379226/