Table of Contents
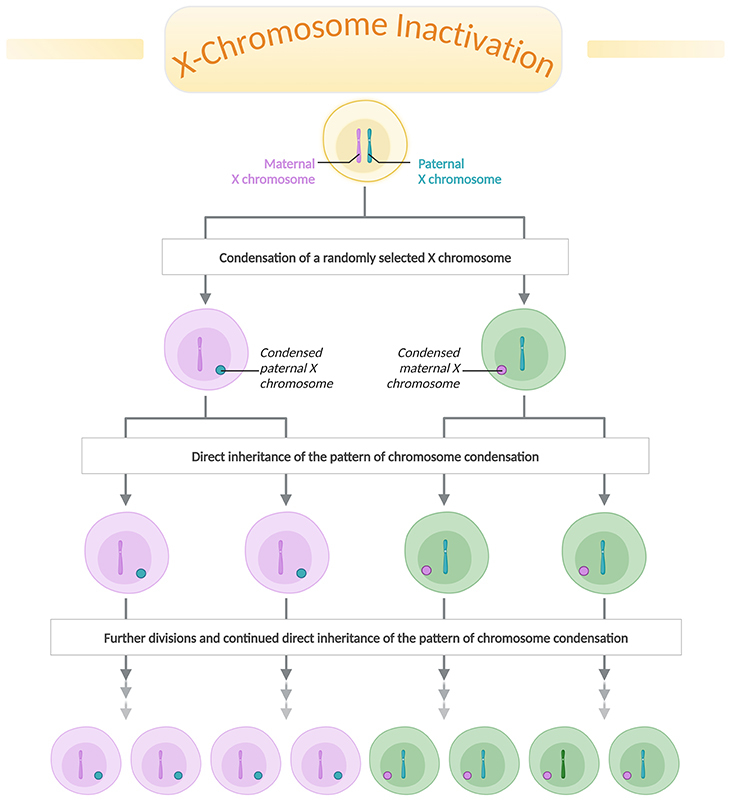
Figure 1. X-chromosome inactivation. X-chromosome inactivation (XCI) is a process in female mammals where one of the two X chromosomes (either maternal or paternal) is randomly silenced during early embryonic development. This ensures dosage compensation, which means equalizing the expression of X-linked genes between males (who have one X and one Y chromosome) and females (who have two X chromosomes). The inactivated X chromosome forms a ‘Barr body’ and remains largely inactive throughout the individual’s life, although some genes can escape inactivation. This process is crucial for balancing the gene expression levels between males and females.
Introduction
The field of epigenetics has revolutionized our understanding of gene regulation, shedding light on how gene expression can be modulated without altering the underlying DNA sequence. This intricate layer of control involves various molecular mechanisms, including DNA methylation, histone modification, and non-coding RNAs. These processes work together to silence or activate genes in a context-dependent manner, profoundly influencing development, physiology, and even behavior.
One of the most striking examples of epigenetic regulation is X-chromosome inactivation (XCI) in female mammals. This process ensures dosage compensation between males and females by randomly silencing one of the two X chromosomes in each female cell, preventing the potential overexpression of X-linked genes. The study of XCI not only provides insights into fundamental biological principles but also exemplifies the complexity and elegance of epigenetic regulation (see Figure 1).
The implications of epigenetics extend far beyond XCI, touching on numerous aspects of health and disease. Epigenetic mechanisms are implicated in various conditions, including cancer, neurological disorders, and developmental abnormalities. Understanding these processes opens new avenues for therapeutic interventions and personalized medicine, highlighting the importance of epigenetic research in contemporary science.
By exploring the mechanics of XCI and the broader field of epigenetics, we can appreciate how molecular interactions shape our biology and influence our lives. This knowledge underscores the dynamic nature of gene regulation, revealing the profound impact of epigenetics on our understanding of genetics and development. (Cf. previous blogs entitled as: “Developmental Origins of Health and Disease: Epigenetics, Nutrition, and Infant Health.” “ Generational Epigenetics: How Nutrition and Environment Shape Lifelong Brain Development.” “ Brain Plasticity – III: Fueling Brain Growth – The Vital Role of Nutrition During Sensitive Periods of Learning.”)
Epigenetic Regulation: The Dynamics of X-Inactivation in Female Development
Understanding Epigenetic X-Inactivation for Everyone: Epigenetics is a fascinating field that explains how our genes can be turned on or off without changing the actual DNA sequence. One of the most interesting examples of this process is X-inactivation, which happens in females. Here’s how it works in a way that everyone can understand:
Why Epigenetics is Important: Think of your DNA as a big recipe book with instructions for everything your body needs to do. Epigenetics is like adding sticky notes to the pages to say, “use this recipe” or “ignore this one.” This is important because it helps our bodies use the right instructions at the right time.
What is X-Inactivation? Women have two X chromosomes (XX) while men have one X and one Y chromosome (XY). To balance things out, women turn off one of their X chromosomes in each cell. This process is called X-inactivation. It’s like having two recipe books but choosing to use only one for each meal. This ensures that women do not have twice as many active X chromosomes as men (see Figure 1).
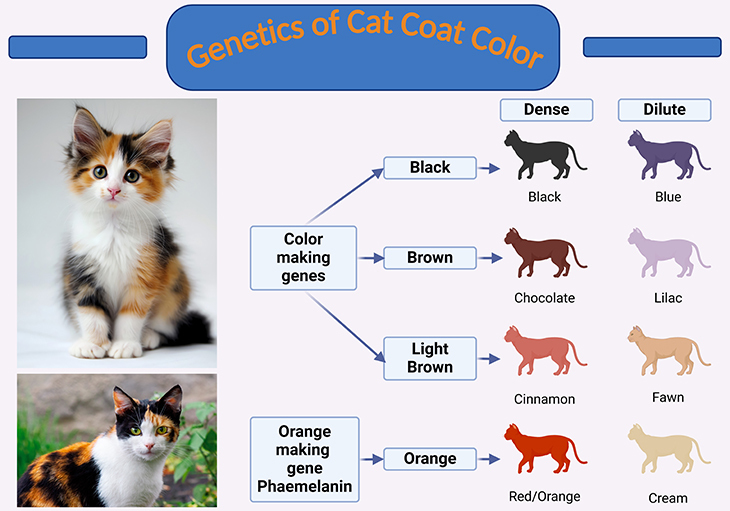
Figure 2. Decoding the genetics behind cat coat colors. The genetics of cat coat color. In cats, eight primary coat colors—black, chocolate, cinnamon, blue, lilac, fawn, red, and cream—result from the interplay of several genes. These colors are produced by pigments called melanins, which originate from specialized cells known as melanocytes. Melanin is deposited in the hair shaft, giving it its color. Three primary genes contribute to the variety of cat coat colors: the color gene, the orange gene, and the color density gene. (1) Color Gene: This gene determines the fundamental color of the coat, which can range from black to brown or light brown. (2) Orange Gene: This gene decides whether the coat will be orange or non-orange. (3) Color Density Gene: This gene influences the distribution of pigment throughout the hair, resulting in either a dense or dilute color. These genetic interactions create the diverse array of coat colors seen in cats, showcasing the complexity and beauty of feline genetics. {For further details: Please cf. E. C. Stubbs, K. Campbell. 2011 Environmental Science, Biology. Why are cloned cats not identical, implications for pet cloning and public perception. Corpus ID: 86249625.}
[https://www.nottingham.ac.uk/biosciences/documents/burn/2011/why-are-cloned-cats-not-identical–emma-stubbs.pdf]
How X-Inactivation Works: Early in a female embryo’s development, each cell randomly decides which X chromosome to turn off [1]. This can be the X chromosome from the mother or the one from the father. As a result, females end up with a mix of cells, some using the mother’s X chromosome and some using the father’s. This random switching creates a mosaic pattern where different cells have different active X chromosomes [2].
The Calico Cat Example: Calico cats are a perfect example of X-inactivation in action. These cats, almost always female, have patches of black, white, and orange fur (see Figure 2). Here’s why:
- Fur Color Genes: The gene for fur color is located on the X chromosome. One X chromosome might have a gene for black fur, and the other might have a gene for orange fur.
- Random Inactivation: In each cell, one of the X chromosomes is randomly turned off. So, some cells will have the gene for black fur active, and others will have the gene for orange fur active.
- Patchy Fur: As the cat grows, cells with the same active X chromosome cluster together (i.e., like clonal expansion or clones of cell), creating patches of different colors. This is why calico cats have their distinct patchy fur.
Stability of X-Inactivation: Once a cell decides which X chromosome to turn off, it sticks with that decision forever. This is why a calico cat’s fur pattern doesn’t change as it gets older. The same thing happens in human females, but because our fur color genes are not on the X chromosome, we do not have visible patchy patterns like calico cats.
Cloning and X-Inactivation: When scientists cloned a calico cat named Rainbow, the clone, named CC (for ‘Carbon Copy’), did not look exactly like Rainbow. This happened because the random process of X-inactivation created different fur patterns, even though CC had the same DNA as Rainbow. This shows that identical DNA does not always lead to identical appearances due to epigenetic effects like X-inactivation (see Figure 3) [3].
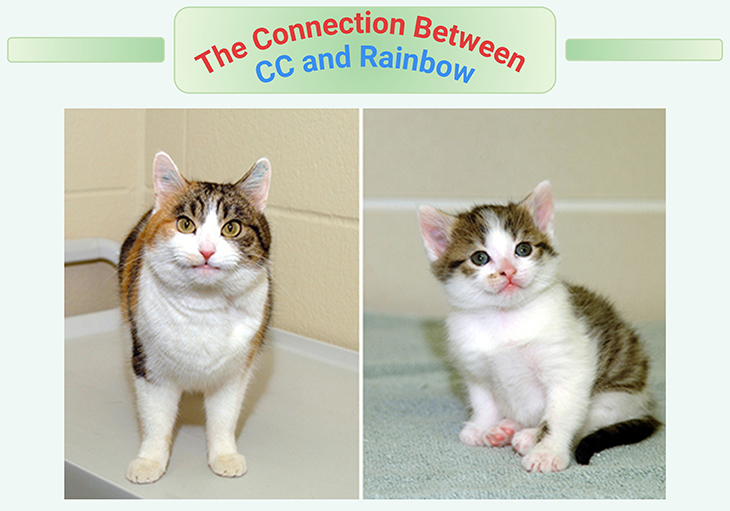
Figure 3. Comparison of calico cats CC and Rainbow. The connection between CC (‘Carbon Copy’) [right image], the first cloned cat, and her genetic donor, ‘Rainbow’ [left image]. Despite having identical genomes, CC and Rainbow exhibit different coat color patterns due to the random nature of X-chromosome inactivation (XCI) in female cats. This epigenetic process silences one of the two X chromosomes in each cell, leading to unique fur color patterns. The phenomenon demonstrates the significant impact of epigenetic regulation on phenotype, resulting in CC not being a perfect replica of Rainbow. {Image and source credits: TAMU, Veterinary Medicine & Biomedical Sciences.} [https://vetmed.tamu.edu/news/press-releases/texas-am-says-goodbye-to-cc-worlds-first-cloned-cat/]
In summary, X-inactivation is a crucial process that ensures balanced gene expression in females by randomly turning off one of their X chromosomes. This process creates unique patterns in animals like calico cats and shows how epigenetics can influence appearance and development.
Epigenetic Silencing: Unraveling the Mechanisms of X-Inactivation
Understanding X-Inactivation: X-inactivation is a process that helps balance gene expression in females by inactivating one of their two X chromosomes in each cell. This process is a key example of epigenetic silencing, where genes are turned off without altering the underlying DNA sequence.
Early Discoveries: In the early 1960s, scientists observed that sometimes a piece of an X chromosome in mice could break off and attach to another chromosome, leading to the inactivation of that chromosome – a phenomenon that normally doesn’t happen. This observation suggested that parts of the X chromosome play a role in its inactivation. Researchers identified a region called the X-inactivation center, which became the focus of intense study [4].
The X-Inactivation Center and XIST: The X-inactivation center produces an RNA transcript called XIST (X-inactive specific transcript) only in X chromosomes that are about to be inactivated. Although the center is present on all X chromosomes, only the ones that will be inactivated express this transcript. XIST is essential for inactivating the X chromosome that generates it. If an X chromosome lacks the critical segments of the X-inactivation center, it cannot be inactivated. Conversely, if these segments are present on other chromosomes, they can cause parts of those chromosomes to be inactivated.
How XIST Works: XIST is a unique RNA that contains signals throughout its length to stop protein translation and never leaves the cell nucleus. Initially discovered in the early 1990s, it was surprising to scientists because it did not seem to be involved in protein construction, which was the known function of RNA at the time. Further research revealed that XIST plays a critical role in epigenetic regulation.
Once expressed, XIST attaches to its X chromosome, covering it and making the genetic information less accessible to the transcription machinery. XIST attracts other molecules to the histones in the region, leading to additional epigenetic modifications that further inhibit transcription. Eventually, the promoters of the genes on the X chromosome become methylated, attracting repressive proteins that help maintain the X chromosome in an inactive state.
The Cascade of Epigenetic Events: Epigenetic regulation often involves a cascade of events where one type of epigenetic change triggers others. DNA methylation can silence genes by preventing transcription machinery from accessing the DNA. Additionally, methyl groups can attract specific proteins that recruit other proteins, including corepressor complexes and enzymes. These enzymes modify histones by removing acetyl groups and adding methyl groups, ultimately closing up the chromatin and deactivating genes. In the case of X-inactivation, DNA methylation and histone modifications together silence most genes on the X chromosome, causing it to become a compact, inactive blob of chromatin in the nucleus, the so called ‘Barr body.’
Implications for Behavioral Epigenetics: For those with a basic understanding of DNA, histones, methyl groups, and related concepts, the new field of behavioral epigenetics becomes surprisingly accessible. The intricate process of X-inactivation illustrates how epigenetic mechanisms can have profound effects on gene expression and development [5-6].
Take-Home Messages
X-Inactivation:
- X-inactivation is a critical epigenetic process in female mammals, ensuring balanced gene expression by randomly silencing one of the two X chromosomes in each cell. This process creates genetic mosaics, as seen in calico cats with their distinct patches of fur color.
Calico Cats:
- The coat patterns in calico cats vividly illustrate the random nature of X-inactivation. Female cats inherit two X chromosomes, each potentially carrying different fur color genes. The random inactivation of one X chromosome in each cell leads to a mosaic of fur colors, making calico cats a practical example of this phenomenon.
Mechanics of X-Inactivation:
- The X-inactivation center (XIC) on the X chromosome produces the XIST RNA, which coats the chromosome and attracts molecules that modify histones and DNA, leading to gene silencing. This cascade of epigenetic events results in a stable, inactive X chromosome, ensuring proper gene dosage between males and females.
Epigenetic Influence:
- The process of X-inactivation highlights the significant role of epigenetics in gene regulation. The presence of XIST RNA and subsequent histone modifications demonstrate how epigenetic mechanisms can profoundly influence phenotype, as evidenced by the different fur patterns in cloned cats like CC and her genetic donor, Rainbow.
Impact on Cloning:
- The case of CC, the first cloned cat, shows that identical genomes can result in different appearances due to epigenetic differences. Despite having the same DNA as her donor, Rainbow, CC’s unique fur pattern emerged from the random X-inactivation process, emphasizing the importance of epigenetic regulation.
These key points provide a cohesive understanding of the role and mechanics of X-inactivation, illustrated through the examples of calico cats and cloned felines.
Summary and Conclusions
X-inactivation is a crucial epigenetic process in female mammals that ensures balanced gene expression by randomly silencing one of the two X chromosomes in each cell, creating genetic mosaics as seen in calico cats. The X-inactivation center (XIC) produces the XIST RNA, which coats the X chromosome and recruits molecules to modify histones and DNA, leading to gene silencing. This cascade of epigenetic changes exemplifies the complexity and importance of epigenetic regulation.
The case of CC (Carbon Copy), the first cloned cat, illustrates that identical genomes can result in different appearances due to epigenetic differences. Despite sharing the same DNA as her genetic donor, Rainbow, CC’s unique fur pattern emerged from the random X-inactivation process, underscoring the significant role of epigenetics in shaping phenotypes.
Understanding the mechanisms of X-inactivation provides valuable insights into epigenetics, a field that studies gene expression changes without altering the DNA sequence. This knowledge extends to behavioral epigenetics, where understanding the roles of DNA, histones, and methyl groups helps us appreciate how gene expression influences behavior and development. Grasping these basic molecular concepts allows us to better understand how our environment and experiences can impact our genes, shaping our health and traits in profound ways. The study of X-inactivation not only enriches our understanding of genetic regulation but also opens new avenues for exploring how epigenetic mechanisms drive complex biological and behavioral outcomes.
For information on autism monitoring, screening and testing please read our blog.
References
- van den Berg IM, Laven JS, Stevens M, Jonkers I, Galjaard RJ, Gribnau J, van Doorninck JH. X chromosome inactivation is initiated in human preimplantation embryos. Am J Hum Genet. 2009 Jun;84(6):771-9. doi: 10.1016/j.ajhg.2009.05.003. Epub 2009 May 28. PMID: 19481196; PMCID: PMC2694969.
https://pubmed.ncbi.nlm.nih.gov/19481196/ - LYON MF. Sex chromatin and gene action in the mammalian X-chromosome. Am J Hum Genet. 1962 Jun;14(2):135-48. PMID: 14467629; PMCID: PMC1932279.
https://pubmed.ncbi.nlm.nih.gov/14467629/
- Holden C. Cloning. Carbon-copy clone is the real thing. Science. 2002 Feb 22;295(5559):1443-4. doi: 10.1126/science.295.5559.1443a. PMID: 11859163.
https://pubmed.ncbi.nlm.nih.gov/11859163/ - Brown CJ, Ballabio A, Rupert JL, Lafreniere RG, Grompe M, Tonlorenzi R, Willard HF. A gene from the region of the human X inactivation centre is expressed exclusively from the inactive X chromosome. Nature. 1991 Jan 3;349(6304):38-44. doi: 10.1038/349038a0. PMID: 1985261.
https://pubmed.ncbi.nlm.nih.gov/1985261/ - Cropley JE, Suter CM, Beckman KB, Martin DI. Germ-line epigenetic modification of the murine A vy allele by nutritional supplementation. Proc Natl Acad Sci U S A. 2006 Nov 14;103(46):17308-12. doi: 10.1073/pnas.0607090103. Epub 2006 Nov 13. PMID: 17101998; PMCID: PMC1838538.
https://pubmed.ncbi.nlm.nih.gov/17101998/ - Cooney CA. Germ cells carry the epigenetic benefits of grandmother’s diet. Proc Natl Acad Sci U S A. 2006 Nov 14;103(46):17071-2. doi: 10.1073/pnas.0608653103. Epub 2006 Nov 13. PMID: 17101997; PMCID: PMC1859889.
https://pubmed.ncbi.nlm.nih.gov/17101997/