Table of Contents
- Introduction
- Epigenetics and Nutrition: How Maternal Diet Shapes Offspring Development
- Epigenetic Influences: How Environment and Experience Shape Gene Expression Across Generations
- Take Home Messages
- Summary and Conclusions
- Did You Know About Folate Receptor Autoantibodies (FRAAs) and Brain Development?
- References
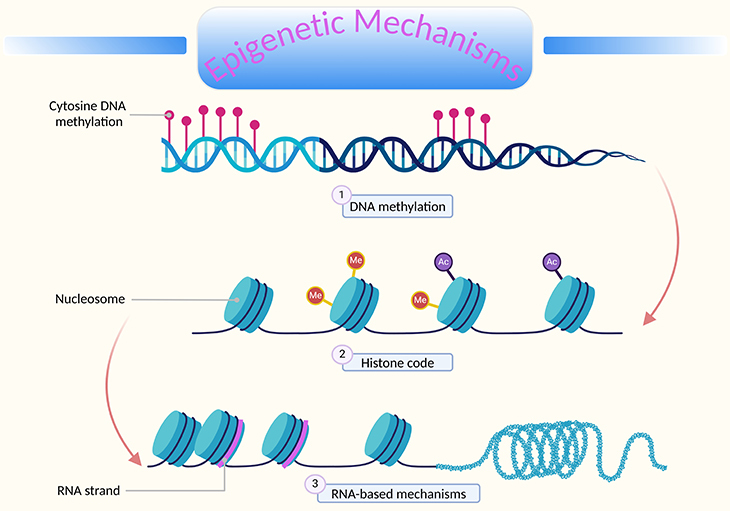
Figure 1. Overview of epigenetic mechanisms. ‘Epigenetics’ literally means ‘above DNA.’ It refers to processes that modify gene expression without altering the underlying DNA sequence. These changes can be passed on during cell division (mitotically inheritable). Key epigenetic mechanisms: (1) DNA methylation – Involves the addition of methyl groups to cytosine nucleotides near gene start sites. Often leads to the repression of gene transcription. (2) Histone modification – Histones (H2A, H2B, H3, H4) are proteins around which DNA is wound. Modifications like acetylation, methylation, and phosphorylation affect how tightly DNA is wound around histones, influencing gene accessibility and expression. (3) RNA-based mechanisms – Involve non-coding RNA molecules, such as microRNAs and long non-coding RNAs. These RNAs can regulate gene expression post-transcriptionally by degrading mRNA or inhibiting its translation. These epigenetic modifications are essential for regulating gene expression and maintaining cellular identity, allowing organisms to adapt to changing environments without altering their genetic code.
Introduction
Our genes, the blueprint of our biological makeup, are not fixed in their function. Instead, they can be influenced by external factors like diet and environment through a process known as epigenetics. This means that what we eat, and our surroundings can cause chemical changes in our DNA, affecting how our genes work without altering the underlying sequence.
Nutrition plays a crucial role in this process. Essential nutrients like folate and choline provide the necessary components for DNA methylation, a key mechanism in gene regulation (see Figure 1). A diet lacking these nutrients, especially during pregnancy, can lead to significant and lasting changes in gene expression, impacting a child’s development and health.
Environmental influences, such as maternal care and prenatal exposure to substances like alcohol, can also cause epigenetic changes. These changes can affect brain development and behavior and can be passed down to future generations. For instance, the amount of care a mother rat provides to her pups can alter their stress response and caregiving behaviors through epigenetic modifications.
Understanding the connection between diet, environment, and epigenetics highlights the importance of a healthy lifestyle and supportive environment, especially during critical developmental periods. These factors together shape our health and well-being, not just for ourselves but for generations to come. (Cf. previous blogs entitled as: “Developmental Origins of Health and Disease: Epigenetics, Nutrition, and Infant Health.” and “Brain Plasticity – III: Fueling Brain Growth – The Vital Role of Nutrition During Sensitive Periods of Learning.”)
Epigenetics and Nutrition: How Maternal Diet Shapes Offspring Development
The epigenetics of nutrition – Our diets influence our epigenetic states because the methyl groups that methylate our DNA come from food. The key molecule in DNA methylation, s-adenosylmethionine (SAM), gets most of its methyl groups from vitamins B2, B6, B9 (folic acid and folate), B12, and choline. Therefore, a diet lacking or abundant in these nutrients can alter the supply of methyl groups (see Figure 1 and 2; Box-1) [1].
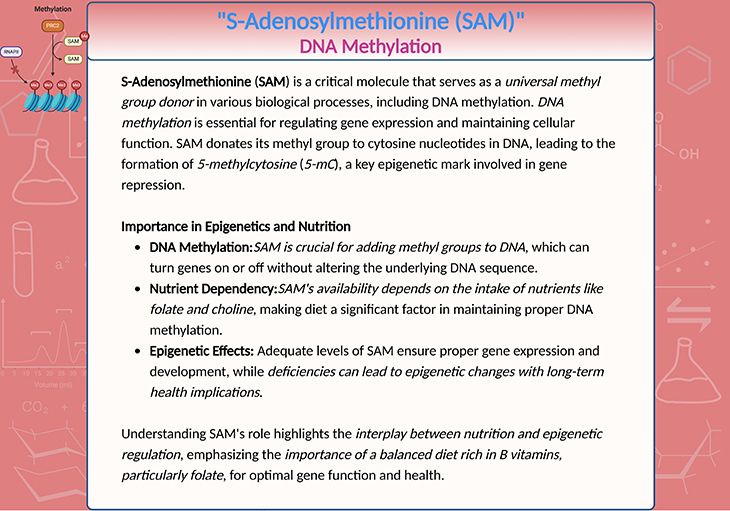
Box-1. S-Adenosylmethionine (SAM) and its significance in epigenetics and nutrition.
These nutrients provide the raw materials necessary for various biological processes, which is why cereals are often fortified with them. For instance, folic acid is vital for fetal development, and doctors advise women who might become pregnant to take folic acid supplements and consume folate-rich foods like eggs, asparagus, liver, and dark leafy greens. A lack of folic acid around conception increases the risk of major congenital abnormalities. Similarly, choline, found in eggs, meats, wheat germ, cauliflower, and milk, is crucial for fetal development and SAM production. Insufficient choline or folate results in low SAM levels, leading to reduced methylation of DNA and histones.
An early study on the effects of maternal diet on offspring’s epigenetics involved feeding pregnant rats a normal diet, a protein-restricted diet, or a protein-restricted diet supplemented with folic acid. The offspring of mothers on the protein-restricted diet had less DNA methylation compared to those of normally fed mothers. Supplementing the diet with folic acid prevented this effect, maintaining normal DNA methylation levels.
Subtle dietary changes during pregnancy can significantly impact offspring. UK scientists fed sheep a methyl-deficient diet for eight weeks before conception and the first week of pregnancy. The offspring, after being transferred to surrogate mothers with complete diets, were fatter, insulin-resistant, and hypertensive as adults [2]. Examination of their fetal DNA revealed altered methylation patterns due to the methyl-deficient diet.
This research suggests that our body compositions may partly result from our mothers’ diets before and during pregnancy. Early nutritional experiences have epigenetic effects seen in humans, rats, and sheep. For instance, a study found that the methylation status of specific DNA segments accounted for about half of the variation in body compositions among nine-year-old children.
Altering choline intake during pregnancy also has long-term effects on offspring’s behavior and neurological traits. Pregnant rats on choline-supplemented diets have offspring with better spatial memory, while choline-deficient diets impair memory performance. These dietary manipulations affect prenatal hippocampus development, influencing learning and memory [3].
Given that a choline-deficient diet reduces access to SAM and alters DNA methylation globally and specifically, it is possible that long-term effects of prenatal nutrition on learning, memory, and body mass result from changes in DNA methylation [5].
Epigenetic Influences: How Environment and Experience Shape Gene Expression Across Generations
Epigenetic mechanisms and sensitive periods – Recent discoveries in developmental genetics reveal that environmental factors can influence gene function across lifespans and even generations, a phenomenon known as epigenetic changes. These changes, or ‘marks,’ are chemical modifications to chromosomes that do not alter the DNA sequence but can significantly impact development and function.
One type of epigenetic mark involves the methylation of cytosine nucleotides near gene start sites, which represses transcription. Other marks include modifications to histone proteins, which can make nearby genes more or less likely to be transcribed (see Figure 1).
A notable example is the role of folate (vitamin B9) in metabolism (see Figure 2). Folate deficiency during pregnancy increases the risk of neural tube defects like spina bifida. The enzyme methionine synthase reductase (MTRR) is essential for folate metabolism. Embryos in the wombs of MTRR mutant mothers exhibit epigenetic defects in DNA methylation, leading to a high incidence of spina bifida. Wild-type embryos transferred to these mutant mothers show epigenetic effects that persist through generations, creating less favorable uterine environments [4].
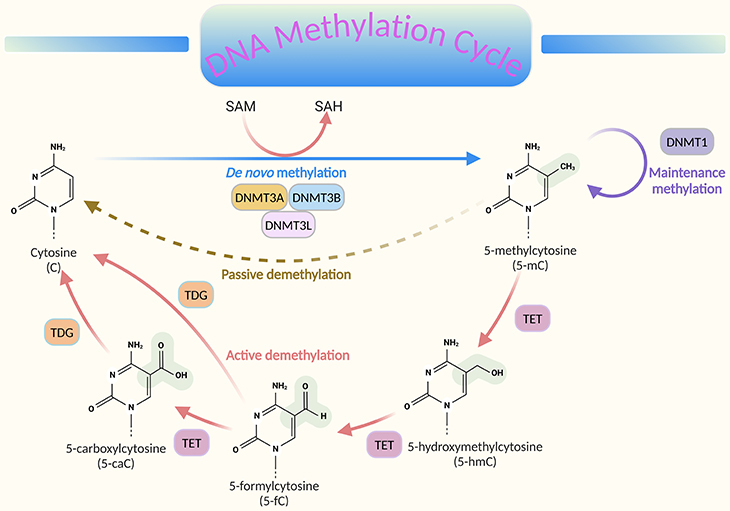
Figure 2. DNA methylation and demethylation cycle. DNA methylation involves the addition of a methyl group (CH3) from the universal donor S-adenosylmethionine (SAM) to the fifth carbon of cytosine (C), facilitated by DNA methyltransferase (DNMT), resulting in 5-methylcytosine (5-mC). This process produces S-adenosylhomocysteine (SAH) as a byproduct. DNA demethylation occurs through passive or active processes: (1) Passive demethylation – Happens over successive cell divisions without the addition of new methyl groups. (2) Active demethylation – Involves the sequential conversion of 5-mC to 5-hydroxymethylcytosine (5-hmC), then to derivatives like 5-formylcytosine (5-fC) and 5-carboxylcytosine (5-caC), ultimately returning to cytosine (C). This process is regulated by the ten-eleven translocation (TET) family of proteins. The combined actions of these mechanisms ensure that DNA methylation and demethylation are dynamic processes, crucial for regulating gene expression and maintaining cellular function.
In humans, high alcohol exposure during pregnancy can cause ‘fetal alcohol syndrome,’ impairing many aspects of brain development. Recent studies link prenatal alcohol exposure to differential methylation of neurodevelopmental genes [6].
Social experiences, such as maternal care, can also lead to epigenetic changes affecting neural development and behavior in subsequent generations. In rodents, maternal behaviors like licking and grooming influence offspring’s stress responses and grooming behaviors through epigenetic modifications. High-grooming mothers produce offspring with lower stress levels and higher grooming tendencies, while low-grooming mothers produce the opposite traits. Cross-fostering experiments confirm that these behaviors are epigenetically inherited.
These epigenetic modifications start when maternal grooming stimulates serotonin release in the hypothalamus, increasing histone acetylase activity in neurons that respond to stress hormones. This leads to demethylation of the glucocorticoid receptor (GR) gene promoter, enhancing its expression and making pups less sensitive to stress. These traits can be reversed by early postnatal experiences, showing the impact of epigenetic marks across generations.
Take-Home Messages
Epigenetics of Nutrition
- Diet Influences Epigenetics: Our diets provide the necessary methyl groups for DNA methylation, crucial for gene regulation.
- Nutrient Deficiency Risks: Deficiencies in key nutrients like folate and choline during pregnancy can lead to long-lasting epigenetic changes, impacting offspring development and health.
- Preventive Nutrition: Supplementing diets with folic acid and other vital nutrients can mitigate negative epigenetic effects.
Epigenetic Mechanisms and Sensitive Periods
- Environmental Impacts: Environmental factors, including maternal care and alcohol exposure, can cause epigenetic changes that affect gene function and behavior across generations.
- Intergenerational Effects: Epigenetic modifications, such as DNA methylation and histone acetylation, can be inherited, influencing the health and behavior of future generations.
- Reversible Marks: Some epigenetic changes can be reversed through early interventions and experiences, highlighting the importance of early life conditions.
Combined Insights
- Holistic Approach: Integrating proper nutrition and favorable environmental conditions during critical developmental periods is essential for optimal cognitive and physical development.
- Long-Term Health: Early nutritional and environmental experiences can have lasting effects on gene expression, underscoring the need for mindful prenatal and early childhood care.
These key points emphasize the profound impact of nutrition and environment on epigenetic mechanisms, shaping gene expression and health outcomes across generations.
Summary and Conclusions
The exploration of epigenetics underscores the profound impact that nutrition and environmental factors have on our genes. These influences can result in chemical modifications that affect gene function, with significant implications for development and health across generations. Adequate nutrition, particularly the intake of B vitamins such as B2, B6, B9 (folate), B12, and choline, is essential for providing the methyl groups necessary for DNA methylation. Folate, in particular, is crucial for preventing neural tube defects during pregnancy and supporting overall fetal development.
Environmental factors, including maternal care and prenatal exposures, also shape gene expression through epigenetic mechanisms. These early influences can lead to lasting changes in behavior, stress responses, and overall health, with effects that can be passed down to future generations.
In summary, a holistic approach to health that includes proper nutrition and a supportive environment during critical developmental periods is vital for optimizing gene function and promoting lifelong well-being. The importance of B vitamins, especially folate, cannot be overstated, as they play a key role in these epigenetic processes. It’s fascinating how interconnected our diet, environment, and genes truly are!
For information on autism monitoring, screening and testing please read our blog.
References
- Zeisel SH. Importance of methyl donors during reproduction. Am J Clin Nutr. 2009 Feb;89(2):673S-7S. doi: 10.3945/ajcn.2008.26811D. Epub 2008 Dec 30. PMID: 19116320; PMCID: PMC2628952.
https://pubmed.ncbi.nlm.nih.gov/19116320/ - Sinclair KD, Allegrucci C, Singh R, Gardner DS, Sebastian S, Bispham J, Thurston A, Huntley JF, Rees WD, Maloney CA, Lea RG, Craigon J, McEvoy TG, Young LE. DNA methylation, insulin resistance, and blood pressure in offspring determined by maternal periconceptional B vitamin and methionine status. Proc Natl Acad Sci U S A. 2007 Dec 4;104(49):19351-6. doi: 10.1073/pnas.0707258104. Epub 2007 Nov 27. PMID: 18042717; PMCID: PMC2148293.
https://pubmed.ncbi.nlm.nih.gov/18042717/ - Craciunescu CN, Albright CD, Mar MH, Song J, Zeisel SH. Choline availability during embryonic development alters progenitor cell mitosis in developing mouse hippocampus. J Nutr. 2003 Nov;133(11):3614-8. doi: 10.1093/jn/133.11.3614. PMID: 14608083; PMCID: PMC1592525.
https://pubmed.ncbi.nlm.nih.gov/14608083/ - Anderson OS, Sant KE, Dolinoy DC. Nutrition and epigenetics: an interplay of dietary methyl donors, one-carbon metabolism and DNA methylation. J Nutr Biochem. 2012 Aug;23(8):853-9. doi: 10.1016/j.jnutbio.2012.03.003. Epub 2012 Jun 27. PMID: 22749138; PMCID: PMC3405985.
https://pubmed.ncbi.nlm.nih.gov/22749138/ - Bekdash RA. Methyl Donors, Epigenetic Alterations, and Brain Health: Understanding the Connection. Int J Mol Sci. 2023 Jan 25;24(3):2346. doi: 10.3390/ijms24032346. PMID: 36768667; PMCID: PMC9917111.
https://pubmed.ncbi.nlm.nih.gov/36768667/ - Laufer BI, Chater-Diehl EJ, Kapalanga J, Singh SM. Long-term alterations to DNA methylation as a biomarker of prenatal alcohol exposure: From mouse models to human children with fetal alcohol spectrum disorders. Alcohol. 2017 May;60:67-75. doi: 10.1016/j.alcohol.2016.11.009. Epub 2016 Nov 22. PMID: 28187949.
https://pubmed.ncbi.nlm.nih.gov/28187949/