Table of Contents
- Introduction
- Neurotypical Brain Structure
- The Cerebral Cortex
- The Subcortical Brain
- Types of Cells in the Human Brain
- Neurons
- Cells that Fire Together, Wire Together
- Cortical Minicolumn
- Glial Cells
- Types of glial cells
- Astrocytes
- Oligodendrocytes and Schwann Cells
- Ependymal cells
- Microglia
- Navigating the Brain
- Caudal and rostral
- Dorsal and ventral
- Medial and lateral
- Superior and inferior
- Conclusion
- Did You Know About Folate Receptor Autoantibodies (FRAAs) and Brain Development?
- References
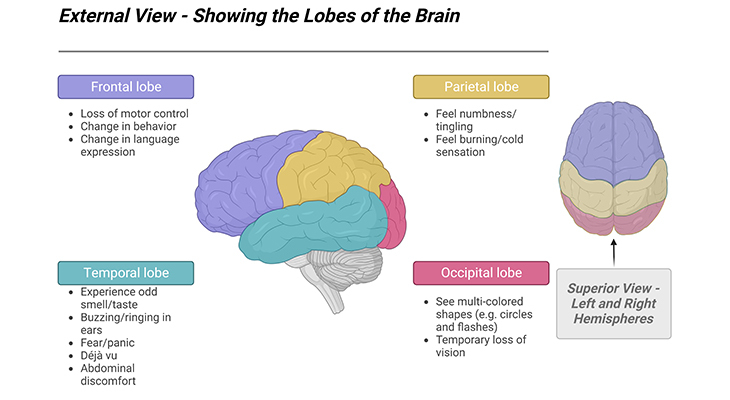
Figure 1. Major Structural Divisions of the Human Brain – External view showing lobes of the brain and superior view revealing both the left and right hemispheres. The brain is divided into two hemispheres, and can be visually divided into four major lobes, viz., frontal, parietal, temporal, and occipital. Although we once thought that these two halves were functionally different, we now know that most of what we do involves both sides of the cerebral hemisphere.
Introduction
The primary aim of this blog and all the subsequent ones are to provide a succinct summary of what is known concerning the neurobiology, or brain bases, of autism, and simultaneously indicate the likely growth areas of research. However, in order to understand the neurobiological bases of autism spectrum disorders (ASD), it is necessary to have some minimal understanding of the neurotypical brain, i.e., some basic facts concerning the structure, function, and development of the neurotypical brain [1-2].
Neurotypical Brain Structure
The human brain is quite amazing! We literally spend all our time living inside it, and we understand the real world we live in because of it. We hear, speak, see, smell, and feel due to its ability to process information. Because of its capacity to generate and process feelings, we laugh, cry, and fall in love. In addition, we are self-aware because of this strange quality called consciousness that goes on somewhere inside our brain. In an incremental fashion, we are gradually adding to our knowledge of how this incredible organ works and, just as important, how it develops, i.e., the developmental process in which the brain grows from a small cluster of cells to brain of a functional adult.
The Cerebral Cortex
The brain and the spinal cord form the central nervous system (CNS). The main part of the human brain, the cerebrum, is divided into two cerebral hemispheres, corresponding to the left and right sides of the body. The hemispheres are joined together by a thick bundles of neural fibers, called the corpus callosum. The hemispheres are symmetrical in as far as they both consist of a frontal, parietal, temporal, and occipital lobe similarly positioned in each hemisphere (Figure 1, 2).
The surfaces of the cerebral hemispheres are enfolded into numerous convolutions (gyri; Greek gyrus = ring or circle) and crevices (sulci; Latin sulcus = furrow) providing a much greater surface area than would otherwise be the case [Box – 1]. Most of these folds are formed by 28 weeks after conception, although they will continue to develop in complexity after birth. The valleys or crevices formed by these main folds are called primary sulci. The biggest of these, the central sulcus, divides the frontal lobe from the parietal lobe.
Box-1
- If you ironed the cerebral cortex flat, it would be a thin 3 mm sheet measuring ≈ 40 cm x 40 cm.
The enfolded brain surface constitutes the cerebral cortex, made of up of grey matter. The cerebral cortex is approximately 2.5 mm thick in humans and is responsible for most the major functions of which we are aware, including many facets of:
- Sensation and motor activity,
- Perception and memory,
- Social ability,
- Language, thought, and reasoning.
There is certainly some specialization within the brain, for instance, for most people language processing is focused primarily in the left temporal region. Nonetheless, the idea that the left brain is the seat of rational thinking and analysis, whereas the right brain is the seat of intuition and emotion, is quite simply, a ‘neuromyth’ [3-4]. There is no scientific evidence whatsoever to support the concept that the right hemisphere is the seat of creative thinking, nor that logical and analytical thinking abilities only utilize the left hemisphere of the brain. In fact, most studies suggest that the hemispheres of the brain do not work separately, but that almost all cognitive tasks use regions of both hemispheres.
The Subcortical Brain
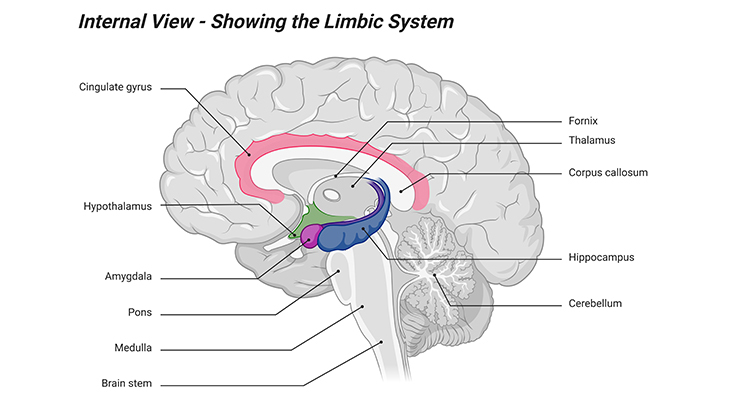
Figure 2. Major Structural Divisions of the Human Brain – Internal View Showing Limbic Structure. The subcortical brain comprises both the brain stem and several other vital parts of the brain that are generally associated with controlling unconscious aspects of various human behaviors.
Beneath the cerebral cortex are the subcortical areas of the brain. The bulk of the cerebral hemispheres is subcortical, subserving functions of which we are less aware and over which we exert less conscious control. These include emotional reactions and behaviors, subserved by structures making up the limbic system (Latin limbus = ring) (Figure 2); also, implicit or unconscious learning of the kinds on which human babies and animals are reliant; also vegetative functions, including:
- Sleep and wakefulness
- Appetite, temperature control
- Some sexual behaviors
At the back of the brain, positioned below the cerebral hemispheres, is the cerebellum, sometimes called the ‘little brain,’ with its own left and right sides and cortical surfaces. Astonishingly, the cerebellum contains about 80% of all the neurons in the human brain [5]. The cerebellum was until relatively recently thought to be involved solely in motor activity (planning and controlling movement). It is now thought to be involved also in various cognitive and social functions, though its precise roles are not well understood, although damage to this region also affects language use and decision-making abilities, so it evidently has many functions.
The structure at the base of the cerebrum is a brain stem, which plays a vital function in regulating reflex activities, for example, respiration, heart rhythm, blood sugar levels. The brain stem also plays a role in relaying information between the brain and spinal cord. In evolutionary terms, the brain stem is the ancient part of the brain, the so-called reptilian brain. Brain stem is composed of medulla, pons, and midbrain; play an important role in regulation and the relay of information (Figure 2).
Types of Cells in the Human Brain
The brains of all species, including the human brain, are primarily composed of two broad classes of cells, viz., neurons and glia or glial cells (Figure 3).
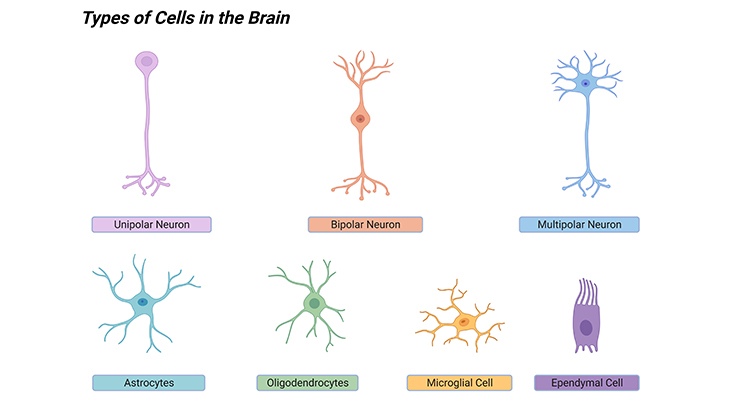
Figure 3. Types of Cells in the Human Brain. The brain contains various types of neurons and glial cells. Neurons fire electrical signals called action potentials, while glial cells perform other cellular functions such as repair and maintenance.
Neurons
Understanding how neurons work is an important aspect of learning how the brain functions. On average, there are 86 billion neurons in the human brain [6], virtually all of them have been generated while the fetus is still in the womb. In the adult brain these neurons are not distributed equally. On average, about 14 to 16 billion neurons are distributed in the cerebral cortex, around 55 to 70 billion of them are in the cerebellum, and about 1 million of them are in the rest of the brain.
Neurons are cells involved in transmitting information in the form of electrical impulses. Clusters of neurons, all of which are involved in transmitting and receiving the same information or signal, are called nuclei (singular: nucleus). The cell bodies are greyish-brown in color, and constitute grey matter in the brain. The cerebral cortex is composed of grey matter, as is much of the cerebellum, there are also clumps of grey matter withing subcortical regions.
Neurons come in three different shapes and sizes, viz., unipolar, bipolar, and multipolar. As well as a central body with a centrally positioned nucleus, the neuron has two types of processes, called axons and dendrites, which extend away from the cell body. Axons are nerve fibers that carry information from one neuron to another, whereas the dendrites (bushy, tree-like fibers) are nerve fibers that act like antennae, receiving the information carried by axons from neighboring cells. The point at which an axon from one neuron junctions with the dendrites of another neuron is called a synapse. There is a very small gap measuring less than a millionth of an inch, called synaptic cleft, between the end of one neuron and the dendrites of the receiving neurons. The synapse converts the electrical activity into a chemical signal (Figure 4).
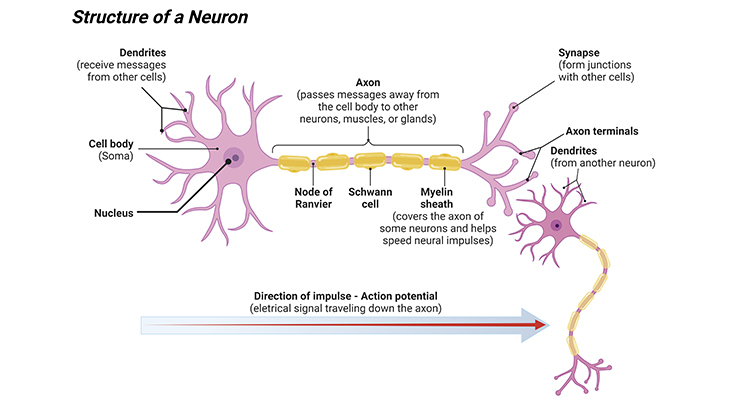
Figure 4. Structure of a Human Neuron – The Fundamental Steps of Neuronal Communication. A typical neuron in the human central nervous system (CNS) receives thousands of inputs at dendritic spines distributed along its dendritic tree. Inputs are collected in the form of synaptic potential, which travel toward the cell body (soma) and are integrated at the axon initial segment to produce action potentials. Action potentials propagate to axon terminals and trigger neurotransmitter release, thus conveying information to postsynaptic partner neurons.
There is a great deal of variety in the size, structure, and appearance of neurons (Figure 3).
- A unipolar neuron has a single branch that extends from the cell body, then divides into two branches. The dendrites extend from one end of the branch and the axons from the other.
- Bipolar neuron has single axon at one end and a single dendrite at the other.
- Multipolar neurons make up most of the neurons in the brain. These have a single axon and many dendrites, in some cases as many as 200,000 dendrites per neuron.
Cells that Fire Together, Wire Together
Neurons fire a very brief electrical charge (a spike, called an action potential) within the length of the axon. The action potential fire when a particular neuron receives sufficient stimulation either from other neurons or from sense receptors such as those in the eye or in the skin that respond to light or to contact/touch, respectively (Figure 4) [Box – 2].
Box-2
OUR BRAIN
“Cells that fire together, wire together”
- 86 billion neurons – (86 x 106)
- Each neuron connects up to 10,000 other neurons – (104)
- Up to 1000 trillion synapses – (1015)
- Nearly 1000 trillion operations per second – (1015)
- About 200 million cortical minicolumns – (2 x 108)
- About 1400 grams – (1.4 Kg)
- 20 Watts power consumption – (20 W)
Cortical Minicolumn
A cortical minicolumn, also known as cortical microcolumn, is a radially oriented network of neurons and fibers which constitutes the smallest module capable of information processing. The cortical minicolumn is a vertical column through the cortical layers of the brain; neurons within the minicolumn receive inputs, have common outputs, are interconnected, and may well constitute a fundamental computational unit of the cerebral cortex [7]. In general, minicolumns consists of around 80 to 120 neurons; and there are about 2 x 108 minicolumns in the human brain. The diameter of a minicolumn is about 28 to 40 μm. Cells in 50 μm minicolumn all have the same receptive field (sensory space); adjacent minicolumns may have different fields. Minicolumns grow from progenitor cells within the embryo and contain neurons within multiple layers (2 to 6) of the cortex.
Glial Cells
The other types of cells in the brain are called glial cells, or simply glia (Greek glia = glue). Glial cells protect, support, and maintain neurons, including forming the myelin sheaths around axons in cases. Glial cells are whiteish in color, and constitute white matter in the brain. There are about the same number of glial cells as there are neurons [5]. Glial cells have a whole range of different cellular functions, for example:
- Support the process of neurotransmission, i.e., transmitting electrochemical signals across the neurons.
- Maintain, especially in balancing the concentration of ions in the spaces between neurons.
- Act to insulate longer neurons to speed up communication.
- Most importantly, processing information during brain development and in later life.
Types of glial cells
- Astrocytes
- Oligodendrocytes and Schwann cells
- Ependymal cells
- Microglia
Astrocytes
Astrocytes are star shaped cells (Greek astron = star) (Figure 3). They keep the brain running by providing energy and the underlying structure to facilitate neurotransmission. When neurons begin to fire, it is the astrocytes which signal to the blood vessels that more oxygenated blood is required. By doing so, they order up, the blood and the glucose it carries to the region of the brain that is most active. In addition, they play an important role in neurotransmission, for example, once a neuron has secreted neurotransmitters and have carried their signal to an adjoining neuron, astrocytes quench the neurotransmitter action and prompt to reabsorb the neurotransmitter back into the neuron. And lastly, astrocytes also have a developmental role, for instance, contributing to the process of forming synapses in the postnatal (after birth) brain [8]
Oligodendrocytes and Schwann Cells
Oligodendrocytes surround the axons in the CNS and form a myelin sheath (Figure 3). The myelin sheath provides a layer of fatty insulation that increases the speed at which electrical signals can propagate more efficiently down the length of the neuron. The process of covering the body of the neuron with insulation is called myelination (Figure 4).
Myelinated cells appear white; and are also referred to as white matter. White matter is the name given to neurons where the axon has been myelinated. On the contrary, grey matter, is a mix of unmyelinated axons, blood vessels, and the synaptic connections between neurons and glial cells.
- The insulation provided by the process of myelination greatly speeds up the action potential as it travels down the axons.
- Myelin does not cover all the axons.
- There are gaps in the covering which occur about once every millimeter.
- These gaps contain the booster stations known as nodes of Ranvier.
- With the help of myelin layer and the nodes of Ranvier – action potential can travel down the axon at the speeds of up to 120 meters per second – that is over 260 mph!
The role performed by oligodendrocytes in the CNS is performed by Schwann cells in the peripheral nervous system (PNS)
Ependymal cells
Ependymal cells are neuroepithelial multiciliated cells that line the ventricular system of the brain and the spinal cord; and are derived from radial glial cells during the initial process of embryogenesis (Figure 3). These cells are involved in the production and secretion of the so-called cerebrospinal fluid (CSF). By beating their cilia, they facilitate the fluid flow (i.e., circulate CSF) and make up the blood-CSF barrier. Thus, ependymal cells play essential roles in human CNS development and normal physiological processes by controlling the production and flow of CSF, brain metabolism, and waste clearance. In addition, they are also thought to act as neural stem cells.
Microglia
Microglia are smaller compared to other glial cells (Figure 3). They are the ‘professional phagocytes of the brain,’ i.e., they are the scavengers within the brain [9]. They act as the first line of defense and respond to injury or disease in the brain; by multiplying and wrapping around damaged neurons and other remaining bits of debris in the brain. They also play a role in pruning unused synaptic connections. They evidently play a vital role in developmental function, considering the fact that practically half of all the cells that are generated during development are eliminated later.
Navigating the Brain
Understanding the language of neuroscience is often quite difficult or puzzling to the non-scientist, in order to navigate the anatomy of the brain, it is important to understand how biologists and neuroscientists describe the relative position of different parts of the body in relation to the spine using the following Latin terms (Figure 5).
Caudal and rostral
In neuroanatomy, caudal implies the tail (Latin caudum = tail) and rostral the nose (Latin rostrum = beak). Since the brain develops in various stages, this can be seen as a hierarchical process. Hence, in neuroscience literature one can sometimes see this described as caudal to rostral development, which simply means that brain development initiates at the bottom and works its way up. As far as the inner parts of the brain, the subcortical regions, develop first, while the cortical regions, the outer parts, develop later.
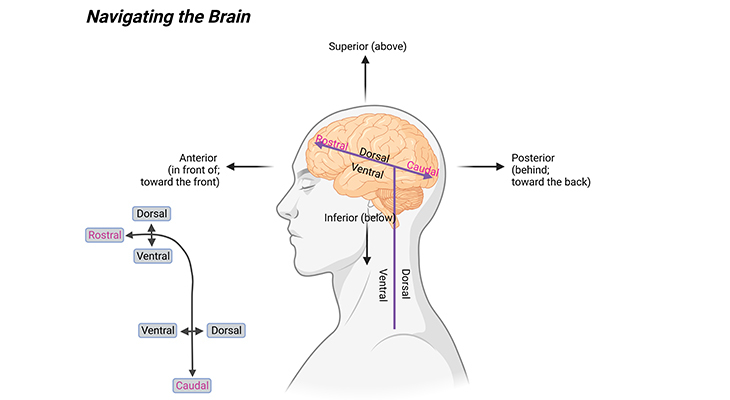
Figure 5. Understanding the language of navigation. (i) Anterior-posterior axis of the CNS, also termed the rostral-caudal axis, meaning snout to tail. (ii) Medial-lateral axis of the CNS, meaning midline to side. (iii) Dorsal-ventral axis of the CNS, meaning back to belly. Note that in humans and other primates, which have curved CNS (inset), some of the anatomical terms may differ from these definitions.
Dorsal and ventral
Dorsal means toward the surface or the top of the head, whereas ventral means towards the surface of the chest or bottom of the head.
Medial and lateral
Medial means towards the middle, while lateral means further away from the middle.
Superior and inferior
Superior denotes above, whereas inferior denotes below.
Conclusion
In this blog we have given a concise account of the structure of the neurotypical brain and described the main functions of different parts of the brain. Nevertheless, it is important to remember that the human brain is a highly complex organ, and all those various regions of the brain are connected by millions of neurons, in an intricate fashion.
For information on autism monitoring, screening and testing please read our blog.
References
- Keenan, T., Evans, S., and Crowley, K. (2016). An Introduction to Child Development. London: Sage.
https://edge.sagepub.com/keenan3e - Hopkins, B., Geangu, E., and Linkenauger, S. (Eds.). (2017). The Cambridge Encyclopedia of Child Development. Cambridge: Cambridge University Press.
https://doi.org/10.1017/9781316216491 - Goswami U. Neuroscience and education: from research to practice? Nat Rev Neurosci. 2006 May;7(5):406-11. doi: 10.1038/nrn1907. PMID: 16607400.
https://pubmed.ncbi.nlm.nih.gov/16607400/ - Goswami U. Neuroscience and education. Br J Educ Psychol. 2004 Mar;74(Pt 1):1-14. doi: 10.1348/000709904322848798. PMID: 15096296.
https://pubmed.ncbi.nlm.nih.gov/15096296/ - Azevedo FA, Carvalho LR, Grinberg LT, Farfel JM, Ferretti RE, Leite RE, Jacob Filho W, Lent R, Herculano-Houzel S. Equal numbers of neuronal and nonneuronal cells make the human brain an isometrically scaled-up primate brain. J Comp Neurol. 2009 Apr 10;513(5):532-41. doi: 10.1002/cne.21974. PMID: 19226510.
https://pubmed.ncbi.nlm.nih.gov/19226510/ - Herculano-Houzel S. (2017). The Human Advantage: A New Understanding of How Our Brain Became Remarkable, Cambridge, MA: MIT Press.
https://mitpressbookstore.mit.edu/book/9780262533539 - Buxhoeveden DP, Casanova MF. The minicolumn hypothesis in neuroscience. Brain. 2002 May;125(Pt 5):935-51. doi: 10.1093/brain/awf110. PMID: 11960884.
https://pubmed.ncbi.nlm.nih.gov/11960884/ - Clarke LE, Barres BA. Emerging roles of astrocytes in neural circuit development. Nat Rev Neurosci. 2013 May;14(5):311-21. doi: 10.1038/nrn3484. Epub 2013 Apr 18. Erratum in: Nat Rev Neurosci. 2013 Jun;14(6):451. PMID: 23595014; PMCID: PMC4431630.
https://pubmed.ncbi.nlm.nih.gov/23595014/ - Wolf SA, Boddeke HW, Kettenmann H. Microglia in Physiology and Disease. Annu Rev Physiol. 2017 Feb 10;79:619-643. doi: 10.1146/annurev-physiol-022516-034406. Epub 2016 Dec 7. PMID: 27959620.
https://pubmed.ncbi.nlm.nih.gov/27959620/