Table of Contents
- Introduction
- Intestinal Hydrolysis of Folate Polyglutamates
- Intestinal Transport of Folate Monoglutamates
- Enterohepatic Circulation of Folates (Transport in- and Storage in- the Liver)
- Clinical Significance
- Conclusion
- Did You Know About Folate Receptor Autoantibodies (FRAAs) and Brain Development?
- References
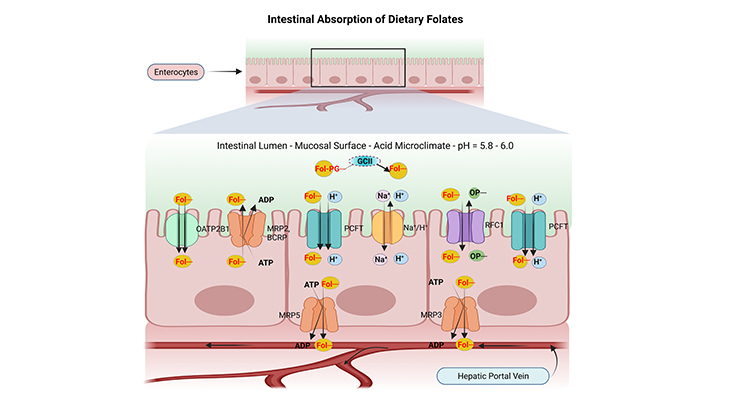
Intestinal absorption of dietary folates is an important, complex, and fascinating process in human metabolism. Folates (Vitamin B9) are critical nutrients that are essential for numerous cellular processes, including cell division and DNA synthesis. The absorption and distribution of folates in the human body involves many intricate steps and we attempt to elucidate this in the following blog.
Figure 1. The Intestinal Absorption and Transport of Dietary Folates. (a) Most dietary folates are polyglutamate derivatives (Fol-PG–) and are hydrolyzed to monoglutamate (Fol–) by the enzyme glutamate carboxypeptidase II (GCII) in the gut before absorption across the intestinal mucosa, and then transported into cells through the proton-coupled folate transporter (PCFT). (b) Both the process of hydrolysis and PCFT-mediated transport have low pH optima and are favored by the low pH within the microenvironment at the jejunal microvilli (green zone) that is generated by Na+/H+ exchangers (Na+/H+). (C) Reduced folate carrier 1 (RFC1) is expressed at the apical brush-border membrane; however, it may not be functional at the pH in this region at usual folate dietary levels. (d) Cellular folates exit through the basolateral membrane by a mechanism that probably involves a member of the multidrug-resistance-associated protein (MRP) family of ATP-binding-cassette (ABC) exporters, for instance, MRP2, 3, 5 and/or breast cancer resistance protein (BCRP/ABCG2). (e) Finally, the folates that are absorbed from the intestine are delivered to the liver via the hepatic portal vein and enter the so-called enterohepatic circulation. (ATP, adenosine triphosphate; ADP, adenosine diphosphate; OP–, organic phosphate gradient; OATP2B1/SLCO2B1, organic anion-transporting polypeptide 2B1).
Introduction
Dietary folates exist predominantly in the polyglutamyl form containing up to seven glutamate residues. Nearly two-thirds of total folate intake from a mixed unfortified diet is in the polyglutamyl form, derived mainly from vegetables [1]. The intestinal absorption of dietary folates is a two-step process that involves (Figure 1, 2):
- The hydrolysis of folate polyglutamates to the corresponding monoglutamyl derivatives.
- Followed by their transport through the intestinal membranes into the enterocytes.
Intestinal Hydrolysis of Folate Polyglutamates
The mechanism by which the dietary folates cross the enterocyte (mucosal cell) and are released across the basolateral membrane into the portal circulation is not fully understood, nevertheless some critical features have been clarified recently (Figure 1).
Hydrolysis of the polyglutamyl chain is an obligatory step in folate absorption because only monoglutamyl forms are able to cross cell membranes. The hydrolysis of polyglutamyl folates occurs in the proximal part of the jejunum with the involvement of the brush-border enzyme viz., glutamate carboxypeptidase II {GCPII or GCII; aka – folate hydrolase, folylpolyglutamate hydrolase, pteroylpolyglutamate hydrolase, pteroylpoly-γ-glutamate carboxypeptidase, or γ – glutamyl hydrolase}.
GCPII acts as an exopeptidase, cleaving terminal γ-linked glutamate residues from polyglutamyl folates in a systematic and progressive stepwise way to release folates with different glutamate chain lengths. GCPII has an optimal pH of 6.5. Research studies have shown that the maintenance of this optimal pH is critical for the complete deconjugation of polyglutamyl folates in the jejunum [2]
(Figure 2).
Remarkable differences have been reported in the activity of intestinal GCPII among different animal species. In general, a high GCPII activity was observed in humans and pigs, whereas a very low to no enzyme was detected in monkeys and rats.
Intestinal Transport of Folate Monoglutamates
Both proton-coupled folate transporter (PCFT) and reduced folate carrier (RFC1) are expressed on the apical membranes of the intestinal mucosa, and their respective mRNAs are upregulated under circumstances of folate depletion. Despite the fact, transport into the mucosal cell appears to occur primarily through the PCFT carrier. The enterocytes mucosal surface is bathed by an acid microclimate. An optimal pH of about 5.5 is required for intestinal folate transport, and folic acid is transported as effectively as reduced folate monoglutamates. All of these are consistent with transport by PCFT carrier. Consequently, RFC1 could not be an effective transporter under these conditions.
PCFT and RFC1 are not expressed on the basolateral membrane of the intestinal mucosal cells, and naturally folate efflux into the portal circulation is apparently believed to be mediated by multidrug-resistance-associated protein (MRPs) family of ATP-binding-cassette (ABC) exporters, for example, MRP3 and/or MRP5, one of the low-affinity, high-capacity transporters [3].
Mechanisms of Folate Absorption Across the Apical Brush-Border Membrane of the Intestinal Mucosa Folate monoglutamates are absorbed in the intestine through saturable and non-saturable transport mechanisms (Figure 2).
The saturable transport operates at the dietary range of folate intake {concentrations < 10 μmol / L} mainly in the duodenum and upper part of jejunum [4]. It is a carrier-mediated process with a pH optimum within the mildly acidic range (pH 6.0). In addition, evidence shows that oxidized and reduced folates are transported with similar affinity. RFC1 and PCFT are considered to be the transmembrane proteins involved in this process.
A nonsaturable transport of folate functions at concentrations of folate within the supraphysiological and pharmacological range of intake {concentrations > 10 μmol / L}, mainly occurring in the ileum without the direct involvement of any specific transporter [5]. At present, there are no data evidently showing that nonsaturable transport is affected by any dietary or physiological factors; consequently, it is considered as an important route for folate absorption in patients with impaired saturable transport, such as hereditary folate malabsorption and celiac disease.
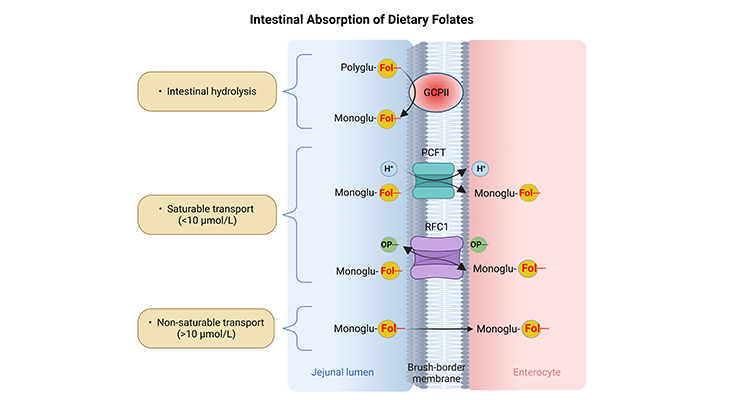
Figure 2. A schematic representation of intestinal hydrolysis and absorption of folates. Step (1) – Intestinal hydrolysis: The hydrolysis of folate polyglutamates (Polyglu-Fol–) to the corresponding monoglutamyl (Monoglu-Fol–) derivatives. Step (2) – Transmembrane transport: Followed by their saturable or non-saturable transport through the intestinal membranes into the enterocytes. (GCPII, glutamate carboxypeptidase II; PCFT, proton-coupled folate transporter; RFC1, reduced folate carrier 1, OP–, organic phosphate gradient.) [Adapted and modified from: Alemdaroglu NC, et al., Biopharma Drug Dispos 2008, 29:335-48. PMID: 18551467]
A variable proportion of plasma folate is bound to low-affinity protein binders, primarily the albumin, which accounts for about 50% of bound folate. This proportion is increased in folate deficiency conditions or syndrome. In addition, plasma also contains low concentrations of a soluble form of the folate receptor (FR). Notably, the levels of this high-affinity, low-capacity binder are elevated in pregnancy and are demonstrably very high in patients suffering from certain leukemias. Generally, increased concentrations of the high-affinity binder may reflect tissue damage or incidental release from tissues, and the effect of plasma protein binding on folate availability for tissues remains as an open question.
Enterohepatic Circulation of Folates (Transport in- and Storage in- the Liver)
Pteroylmonoglutamates, primarily 5-methyl-tetrahydrofolate (5-methyl-THF), are the circulating forms of folates in plasma, moreover, mammalian cells are not believed to transport polyglutamates of chain length three and above. After folate absorption into the portal circulation, much of this folate can be taken up by the liver, where it is either:
- Metabolized to polyglutamate derivatives and retained, or
- It is released into blood or bile.
An enterohepatic circulation of folate has been described involving release of hepatic 5-methyl-THF into bile via MRP2 with reabsorption in the small intestine. The preponderance of 5-methyl-THF in plasma probably reflects that this is the major cytosolic folate in mammalian tissues and consequently is the major form that would be released. The extent, if any, of the release of short chain folylpolyglutamates from tissues is unknown. Plasma contains γ-glutamyl hydrolase (γ-GH/GCPII) activity, and any polyglutamate released into plasma would be hydrolyzed into the monoglutamate [6].
To summarize, folates that enter the liver have three potential destinations as follows [3]:
- Folates can be converted into polyglutamate storage forms, or
- They can be secreted into the bile at the hepatic canalicular membrane mediated by MRP2, whereby they return to the duodenum and jejunum and are subsequently reabsorbed, thus completing the cycle of enterohepatic circulation, or
- Folate monoglutamates, formed by hydrolysis of polyglutamate forms stored in hepatocytes, or delivered directly from the hepatic portal vein, can enter the hepatic vein, ultimately reaching the systemic circulation whereby they accumulate in and meet the one carbon requirements of, peripheral tissues.
Box-1
- Plasma folate concentrations in humans are usual in the 10 to 30 nM range, whereas hepatic (liver) levels, practically all polyglutamates, are reported to range from about 10 to 30 μM.
- Total body stores of folate in humans have been estimated to range from 10 to 100 mg, with 3 to 16 mg in liver.
Clinical Significance
- Hereditary Folate Malabsorption (HFM)
HFM is a rare autosomal recessive disorder caused by loss-of-function mutations in the PCFT gene, resulting in systemic folate deficiency and impaired transport of folate into the brain with very low folate levels in the cerebrospinal fluid (CSF) [7, 8]. CSF folate concentrations are normally higher than in plasma. Symptoms develop several months after birth and that include mainly:
- Macrocytic anemia
- Developmental defects or delays
- Seizures
Infants can be treated with high doses of oral folates such as methyl-THF or with low much smaller doses of parental folate, suggesting that some intestinal transport can occur through RFC1 carrier, but also revealing that PCFT is normally responsible for the bulk of folate transport in the intestine. Thus, normalization of CSF folate levels in subjects with HFM disorder requires very high plasma folate concentrations [9].
Conclusions
In a nutshell, here we have highlighted some essential features that govern the intestinal absorption and transport of dietary folates; and addressed simultaneously a number of critical questions pertaining to intestinal absorption of dietary folates, for instance: (1) What is mechanism(s) of folate absorption across the apical brush-border membrane of the intestine under physiological conditions? (2) What role could PCFT, RFC1, and potentially other transporters play? (3) Where are folates absorbed within the small intestine in the presence or absence of PCFT? (4) Which MRP protein(s) is required for transport of folates across the serosal membrane of the human intestine? And finally, (5) How folate is transported and stored in liver, including the so-called enterohepatic circulation of folates.
To summarize, food folates (polyglutamate derivatives) are hydrolyzed to monoglutamate forms in the gut before absorption across the intestinal mucosa. The cleavage is accomplished by the enzyme GCPII (γ-GH). The monoglutamate form of folate is actively transported across the proximal small intestine by a saturable pH-dependent process. When pharmacological doses of the monoglutamate form of folate are consumed, it is also absorbed by a non-saturable mechanism involving passive diffusion.
Monoglutamates, mainly 5-methyl-THF, are present in portal circulation. Much of this folate can be taken by the liver, where it is metabolized to polyglutamate derivatives and retained or released into the blood or bile. Approximately two-thirds of the folate in plasma is protein bound.
For information on autism monitoring, screening and testing please read our blog.
References
- Halsted CH. The intestinal absorption of dietary folates in health and disease. J Am Coll Nutr. 1989 Dec;8(6):650-8. doi: 10.1080/07315724.1989.10720340. PMID: 2695555.
https://pubmed.ncbi.nlm.nih.gov/?term=halsted+ch+1989 - Chandler CJ, Wang TT, Halsted CH. Pteroylpolyglutamate hydrolase from human jejunal brush borders. Purification and characterization. J Biol Chem. 1986 Jan 15;261(2):928-33. PMID: 2867095.
https://pubmed.ncbi.nlm.nih.gov/2867095/ - Zhao R, Matherly LH, Goldman ID. Membrane transporters and folate homeostasis: intestinal absorption and transport into systemic compartments and tissues. Expert Rev Mol Med. 2009 Jan 28;11:e4. doi: 10.1017/S1462399409000969. PMID: 19173758; PMCID: PMC3770294.
https://pubmed.ncbi.nlm.nih.gov/19173758/ - Rosenberg IH, Zimmerman J, Selhub J. Folate transport. Chemioterapia. 1985 Oct;4(5):354-8. PMID: 3907863.
https://pubmed.ncbi.nlm.nih.gov/3907863/ - Washington (DC): National Academies Press (US); 1998. Dietary Reference Intakes for Thiamin, Riboflavin, Niacin, Vitamin B(6), Folate, Vitamin B(12), Pantothenic Acid, Biotin, and Choline. Institute of Medicine (US) Standing Committee on the Scientific Evaluation of Dietary Reference Intakes and its Panel on Folate, Other B Vitamins, and Choline et al.
https://www.ncbi.nlm.nih.gov/books/NBK114310/?term=Dietary%20Reference%20Intakes%20for%20Thiamin%2C%20Riboflavin%2C%20Niacin%2C%20Vitamin%20B6%2C%20Folate%2C%20Vitamin%20B12%2C%20Pantothenic%20Acid%2C%20Biotin%2C%20and%20Choline%20%2C%2020232 - Visentin M, Diop-Bove N, Zhao R, Goldman ID. The intestinal absorption of folates. Annu Rev Physiol. 2014;76:251-74. doi: 10.1146/annurev-physiol-020911-153251. PMID: 24512081; PMCID: PMC3982215.
https://pubmed.ncbi.nlm.nih.gov/24512081/ - Geller J, Kronn D, Jayabose S, Sandoval C. Hereditary folate malabsorption: family report and review of the literature. Medicine (Baltimore). 2002 Jan;81(1):51-68. doi: 10.1097/00005792-200201000-00004. PMID: 11807405.
https://pubmed.ncbi.nlm.nih.gov/11807405/ - Qiu A, Jansen M, Sakaris A, Min SH, Chattopadhyay S, Tsai E, Sandoval C, Zhao R, Akabas MH, Goldman ID. Identification of an intestinal folate transporter and the molecular basis for hereditary folate malabsorption. Cell. 2006 Dec 1;127(5):917-28. doi: 10.1016/j.cell.2006.09.041. PMID: 17129779.
https://pubmed.ncbi.nlm.nih.gov/17129779/ - Goldman ID. Hereditary Folate Malabsorption. 2008 Jun 17 [updated 2022 May 5]. In: Adam MP, Mirzaa GM, Pagon RA, Wallace SE, Bean LJH, Gripp KW, Amemiya A, editors. GeneReviews® [Internet]. Seattle (WA): University of Washington, Seattle; 1993–2023. PMID: 20301716.
https://pubmed.ncbi.nlm.nih.gov/20301716/