Table of Contents
- Introduction
- Microbiomes, the Brain, and Behavior
- Nervous System and its Role in Behavior
- Gut-Brain Axis
- Microbial Products and Brain Function
- The “Happiness Hormone” Serotonin
- The Gut Microbiome and the Brain
- The Role of Vagus Nerve in the Microbiome-Gut-Brain Axis
- The Role of Immune System in the Microbiome-Gut-Brain Axis
- The Intricate Role of Macrophages in the Microbiome-Gut-Brain Axis
- Role of Nutrition and Metabolism in Microbiome-Gut-Brain Axis
- The Role of Maternal Microbiota in Fetal and Offspring Neurodevelopment
- Take Home Messages
- Summary and Conclusions
- Did You Know About Folate Receptor Autoantibodies (FRAAs) and Brain Development?
- References
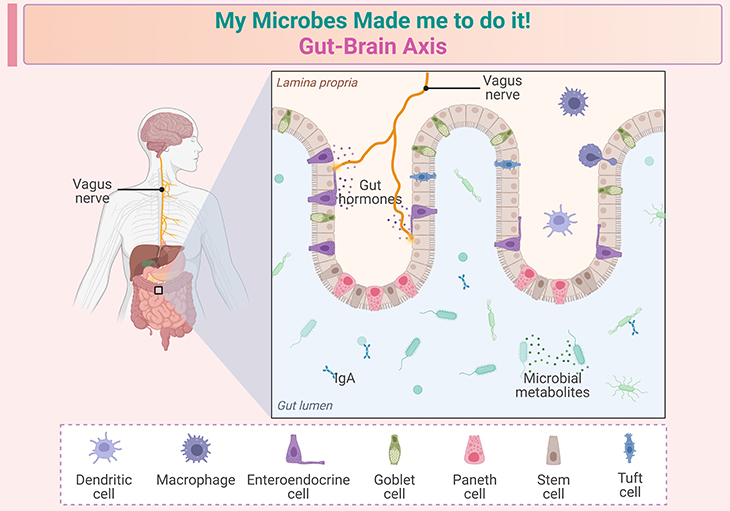
Figure 1. My microbes made me to do it! ~ The gut-brain axis. The role of the gut microbiome in early childhood development is a rapidly growing area of research. Here are some key points based on recent studies: (a) Critical windows in early life: the first few years of life are crucial for both microbiome and brain development. During this period, the gut microbiome can significantly influence neurodevelopmental processes. Studies have shown that disruptions in the gut microbiome during these critical windows can lead to long-term effects on brain function and behavior. (b) Neurodevelopmental disorders: there is growing evidence linking gut microbial dysfunction to various neurodevelopmental disorders, such as autism spectrum disorder (ASD) and attention-deficit/hyperactivity disorder (ADHD). For instance, children with ASD often exhibit altered gut microbiota composition compared to neurotypical children. (c) Mechanisms of influence: the gut microbiome can affect brain development through several mechanisms, including modulation of the immune system, production of neuroactive metabolites, and direct neural communication via the vagus nerve. These interactions can influence processes such as neurogenesis, myelination, and synaptic pruning. (d) Potential interventions: understanding the role of the gut microbiome in early neurodevelopment opens up possibilities for interventions. Probiotics, prebiotics, and dietary modifications are being explored as potential strategies to support healthy brain development and mitigate the risk of neurodevelopmental disorders. These insights highlight the importance of maintaining a healthy gut microbiome during early childhood to support optimal brain development.
Introduction
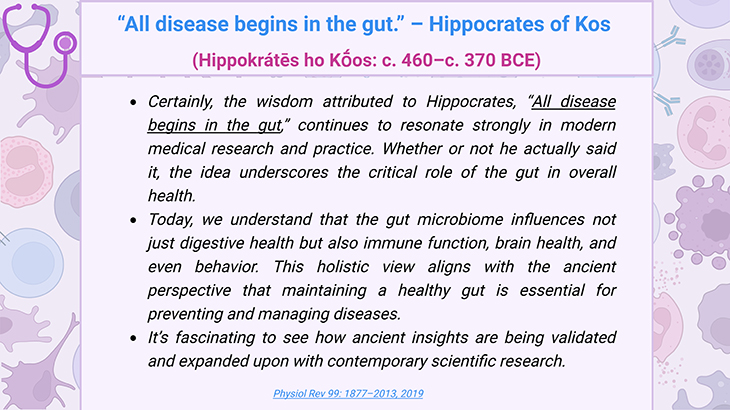
Box-1. All disease begins in the gut ~ Hippocrates of Kos. (Mood by microbe: towards clinical translation. Dinan and Cryan 2016 Genome Medicine 8:36: 1–3) [1].
‘My microbes made me do it!’ Until now, this assertion would have sounded like a pretext of last resort, and any indications that the gut microbiome may influence our behavior would have been discounted as fanciful speculation. Recent research has certainly shown that the gut microbiome can significantly influence our behavior, mood, and cognitive functions (learning, memory, and emotional state). This is primarily due to the ‘gut-brain axis,’ a complex communication network that links the gut and the brain through biochemical and physical pathways (see Figure 1; Box-1; Box-2).
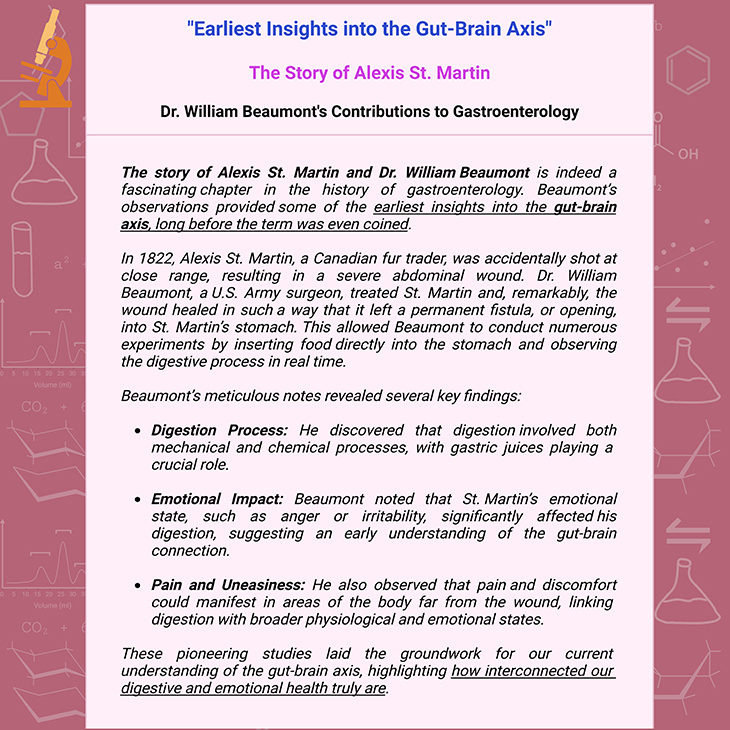
Box-2. Earliest insights into the gut-brain axis ~ The story of Alexis St. Martin and Dr. William Beaumont. Dr. William Beaumont’s meticulous observations of the digestive process in real time. (Cryan et al., 2019 Physiol Rev 99: 1877–2013) [2].
Gut microbes produce neurotransmitters, hormones, and metabolites that can affect our emotions, thought processes, and behaviors. For example, the gut produces a large portion of the body’s serotonin, a neurotransmitter that plays a key role in mood regulation. Studies have shown that changes in the gut microbiome can lead to alterations in brain function and behavior, as seen in experiments with laboratory mice and fruit flies.
Nonetheless, given the evolutionary history of interactions between animals and their resident microorganisms, it is reasonable to expect that these findings are relevant to humans as well. This area of research is still evolving, but it holds promising potential for understanding and possibly treating various mental health conditions through modulation of the gut microbiome.
Microbiomes, the Brain, and Behavior
What is Behavior
Behavior can be defined as the coordinated response of an animal to external or internal stimuli. The following examples of the cat and mouse illustrate how behavior can be driven by both external and internal stimuli, and how these can interact.
- a cat may chase a mouse that it sees (response to an external stimulus),
- forage for mice when it is hungry (response to an internal stimulus), or
- only chase the mouse when it is hungry (combined response to external and internal stimuli).
Many biologist and most psychologists would add a proviso to this definition ; that
behavior has evolved to have consequences, to effect either a change in the relationship of the organism with its environment (the cat catches the mouse) or to maintain the status quo (the mouse escapes). This interaction is a key aspect of understanding animal behavior, including humans. Thus, the idea that behavior has evolved to have consequences is crucial, as it underscores the role of behavior in survival and adaptation.
Moreover, behavior does not necessarily involve movement. Cognitive processes, such as planning your activities for the day, are indeed behavior with significant consequences, even though they may not involve physical action (no movement), for example, blinking.
Nervous System and its Role in Behavior
The biological substrate for animal behavior is the nervous system, including the brain. The essential functional unit of the nervous system is the neuron (also known as nerve cell; a nerve is made up of many neurons). The nervous system transmits information very rapidly, by electrical signals along the length of each neuron (axons) and by chemical signals (neurotransmitters) related at specialized sites, called synapses, between neurons.
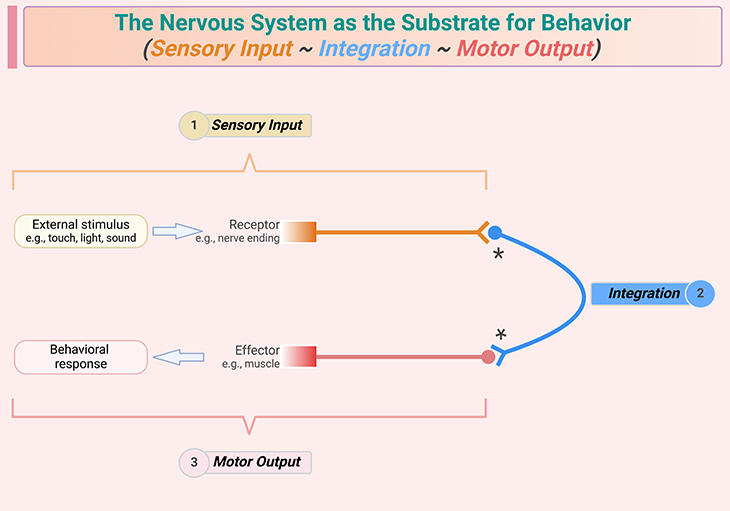
Figure 2. The nervous system as the substrate for behavior. The three-step process comprises: (1) sensory input, (2) integration of the sensory inputs and the internal state of the animal, lead to (3) a behavioral response, which usually involves movement of the animal, i.e., motor output. Note that integration ranges in complexity from a single relay neuron (as shown) to complex patterns of signaling that involve multiple regions of the brain and sophisticated evaluation of information, generating a coordinated behavioral response; and some behavioral outputs do not involve movement.
The basis of behavior is a three-step process: sensory input, central integration, and the behavior response, which usually involves motor output (see Figure 2). The integration step can be highly sophisticated, especially in organisms with flexible or complex behaviors, and it can involve large numbers of neurons in multi-way communication via synaptic connections that stimulate or depress the electrical activity of other neurons. In most animals, the central site of integration is the brain. The human brain contains approximately 100 billion (1011) neurons, each of which estimated to have, on average, 1,000 synapses with other neurons.
To recapitulate, the three-step process of sensory input, central integration, and behavioral response is fundamental to understanding how organisms interact with their environment. The sheer number of neurons and synapses in the human brain highlights the incredible capacity for processing and integrating information.
Gut-Brain Axis
There is now accumulating evidence that the gut microbiome can influence nervous system function, and particularly the central integration that dictates the behavioral response to internal and external stimuli. At a glance, this appears biologically far-fetched because the gut microbiome is remote from the brain. However, interactions between the gut microbiome and brain function are a mere extension to the long-recognized physiological relationship between the brain and gut, this connection is primarily mediated by several pathways, including the nervous, endocrine, and immune system: the so called gut-brain axis (see Figure 1).
Gut microbes produce neurotransmitters and other chemicals that can affect brain function. For instance, they help produce serotonin, a key neurotransmitter involved in mood regulation. Additionally, the vagus nerve, which runs from the gut to the brain, plays a crucial role in this bidirectional communication. Research has shown that changes in the gut microbiome can influence stress responses, anxiety, and even cognitive functions like memory. This is why maintaining a healthy gut microbiome is increasingly seen as important for overall mental health and well-being.
One does not have to be a physiologists to appreciate how emotional state, including stress, influences appetite and gut function, as well as how eating a nourishing meal can promote our sense of wellbeing.
Microbial Products and Brain Function
How do microorganism in the gut affect brain function? The simple answer is that (see Figure 4):
- Chemical release: gut microorganism release various chemicals, including neurotransmitters like serotonin, dopamine, and gamma-aminobutyric acid (GABA). These chemicals can influence mood, anxiety, and overall brain function.
- Cell wall components and metabolic waste: cell components of microbial cell walls (that are shed from both proliferating and dying microbial cells), and metabolic waste products can enter the bloodstream and affect distant organ, including brain.
There is more complicated answer to the question about how gut microorganisms influence brain function (see Figure 4).
- Indirect interactions: most microbial chemicals do not directly interact with brain neurons. Instead, they influence other cells, such as:
- Endocrine cells: these cells in the gut wall produce hormones that can affect brain function.
- Immune cells: microbial chemicals can modulate immune responses, which in turn can influence brain activity.
- Neuroactive compounds: gut bacteria produce neuroactive compounds that regulate emotions and cognitive functions.
In the next section, we present some of the patterns of interactions by addressing some widely held claims about how gut microbe-brain interactions affect the so-called happiness hormone.
The “Happiness Hormone” Serotonin
Serotonin is a small molecule that packs a big punch. It is an evolutionarily ancient molecule found across all domains of life. While typically regarded as neurotransmitter, serotonin serves a diverse range of roles across disparate biological systems [3].
It is best known for its role in supporting the feelings of well-being. Depression is associated with low serotonin levels in the brain and various anti-depressant medications function to boost serotonin levels (see Figure 3). The popular press and internet are flooded with narratives that the gut microbiome promotes happiness via its effect on brain serotonin levels. These accounts are based on two fundamental misconceptions.
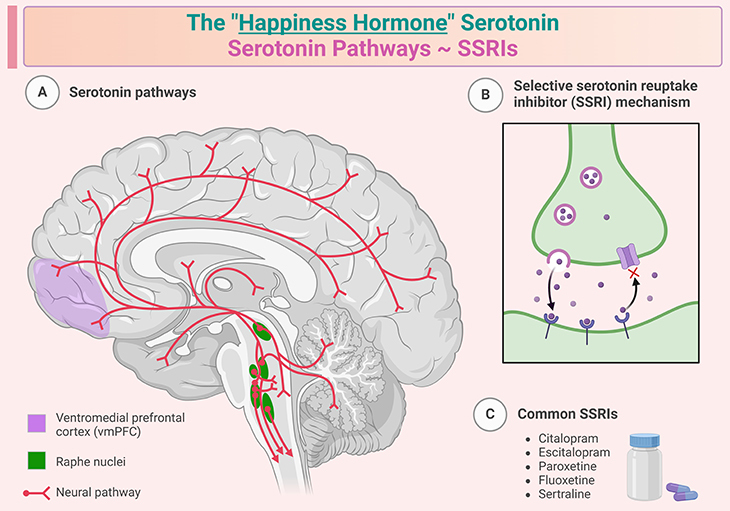
Figure 3. The “happiness hormone” ~ Serotonin. Serotonin, also known as 5-hydroxytryptamine (5-HT), is a neurotransmitter and hormone that helps transmit signals between nerve cells. It is primarily produced in the gastrointestinal tract (about 90%) and in the brain (about 10%) from the amino acid tryptophan. Serotonin plays a crucial role in regulating mood, sleep, digestion, appetite, blood clotting, and bone health. It is often referred to as the “feel-good” chemical because it contributes to feelings of well-being and happiness. Low levels of serotonin are associated with depression and anxiety. In the gut, serotonin helps control bowel movements and function. It also plays a role in reducing appetite while eating. Serotonin is involved in regulating the sleep-wake cycle and is a precursor to melatonin, a hormone that regulates sleep. Serotonin reuptake inhibitors (SSRIs) work by blocking the reabsorption (reuptake) of serotonin into neurons, making more serotonin available to improve transmission of messages between neurons. They are called selective because they primarily affect serotonin and no other neurotransmitters. SSRIs are commonly prescribed to treat depression, anxiety disorders, panic disorder, obsessive-compulsive disorder (OCD), post-traumatic stress disorder (PTSD), and other mood disorders. Some widely used SSRIs include fluoxetine (Prozac), sertraline (Zoloft), citalopram (Celexa), escitalopram (Lexapro), and paroxetine (Paxil). Common side effects of SSRIs can include nausea, insomnia, dizziness, dry mouth, and sexual dysfunction. They are generally considered to have fewer side effects compared to other types of antidepressants. SSRIs are considered relatively safe and effective for long-term use in treating various mental health conditions.
Let us break down these misunderstandings further:
- Serotonin production in the gut: The first misconception is the erroneous claim that gut microorganisms produce most of the serotonin in our bodies. It is accurate that about 90% of the body’s serotonin is produced in the gut. However, this serotonin is primarily synthesized by specialized endocrine cells (enterochromaffin cells, ECCs) in the gut lining, not by gut microbes. While some gut bacteria can produce serotonin as a byproduct, their contribution is minimal compared to these enterochromaffin cells.
- Serotonin and the blood-brain barrier (BBB): The second misconception is the incorrect belief that gut-derived serotonin (whether from microbes or endocrine cells) contributes directly to the levels of serotonin in the brain. It is factual that serotonin produced in the gut does enter the bloodstream and affects various bodily functions, but it does not cross the blood-brain barrier (BBB). The brain’s serotonin is synthesized locally within the brain itself. This is crucial because the BBB restricts most blood-borne compounds, including serotonin, from entering the brain.
Focusing on the scientific facts, we know with confidence that:
- endocrine cells in the gut are stimulated to synthesize and release serotonin by various microbial products, for example, indole, tyramine, and secondary bile acids have been implicated;
- endocrine cell-derived serotonin has important functions, but these functions are not directly related to behavior;
- it activates neurons in the gut wall, stimulating gut muscle to move food along the gut;
- it is also released into the blood, where it promotes efficient blood clotting after injury; and
- supports healthy bone development and immunity.
Therefore, in essence, although the gut microbiome does not contribute directly to the serotonin levels in the brain, various behavioral and neurophysiological studies suggest strongly that the gut microbiome can contribute to emotional health. Some of the underlying mechanisms for this and other behavioral traits are addressed subsequently below.
The Gut Microbiome and the Brain
The gut microbiome indeed plays a crucial role in influencing behavior through its interactions with various physiological systems, including the nervous system, immunity, and metabolism (see Figure 4). Here are some key points based on recent research:
The Role of Vagus Nerve in the Microbiome-Gut-Brain Axis
The first and most obvious route for gut microbiome communication with the brain is one that requires a neural connection between the gut and the brain, i.e., the vagus nerve.
- Neural connection via the vagus nerve: The vagus nerve is a primary pathway for communication between the gut and the brain (and other organs, including the liver, lungs, and heart). Studies have shown that mice with lactobacilli bacteria exhibit reduced anxiety and increased sociability. However, these effects are lost when the vagus nerve is severed, indicating its critical role in mediating these benefits.
- Metabolic interactions: Gut bacteria produce various metabolites that can influence host metabolism and, consequently, brain function. These metabolites can affect the production of neurotransmitters and other signaling molecules. The interaction between gut microorganisms and the vagus nerve is indirect; endocrine cells in the gut wall respond to fermentation products released from the lactobacilli by signaling to nearby nerve endings of the vagus.
These interactions emphasize the complexity and importance of the gut microbiome in regulating not just physical health but also mental and emotional well-being.
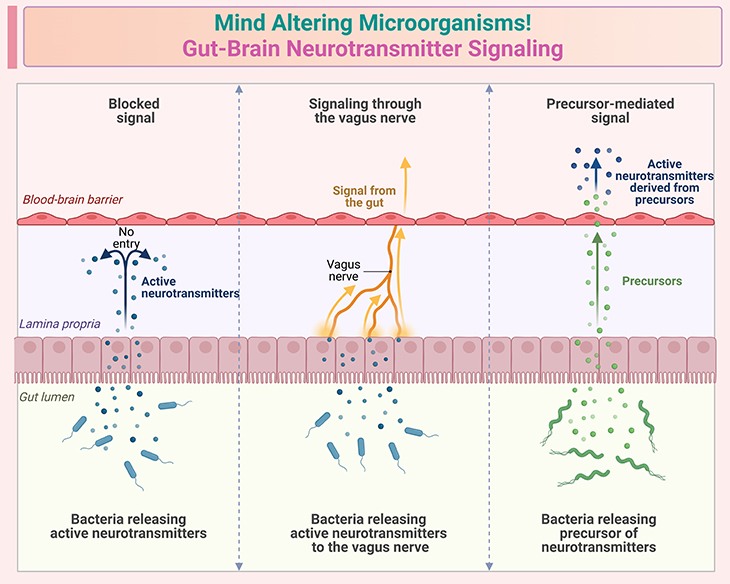
Figure 4. Mind altering microorganisms ~ Gut-brain neurotransmitter signaling. Illustration depicts some established facts about gut-brain neurotransmitter signaling: (i) Bidirectional communication – the gut-brain axis involves two-way communication between the gastrointestinal tract and the central nervous system. This communication occurs through neural, hormonal, and immune pathways; (ii) Neurotransmitters in the gut – the gut produces several neurotransmitters, including serotonin, dopamine, and gamma-aminobutyric acid (GABA). About 90% of the body’s serotonin is produced in the gut. (iii) Vagus nerve: the vagus nerve is a critical component of the gut-brain axis, facilitating direct neural communication between the gut and the brain. It plays a key role in transmitting signals related to gut health and function. (iv) Microbial metabolites – gut bacteria produce metabolites such as short-chain fatty acids (SCFAs), which can influence brain function. These metabolites can cross the blood-brain barrier and affect neurotransmitter systems. (v) Impact on mood and behavior – changes in gut microbiota composition can influence mood and behavior. For example, certain gut bacteria can produce metabolites that affect the production and regulation of neurotransmitters involved in mood regulation; (vi) Neuroendocrine signaling – the gut-brain axis includes neuroendocrine signaling, where hormones released by the gut can influence brain function. This includes hormones like ghrelin and leptin, which regulate appetite and energy balance. (vii) Immune system Involvement – the immune system is also a key player in gut-brain communication. Cytokines and other immune signaling molecules produced in the gut can affect brain function and behavior. These points highlight the complex and dynamic nature of gut-brain neurotransmitter signaling, emphasizing its importance in maintaining overall health and well-being.
The Role of Immune System in the Microbiome-Gut-Brain Axis
The second important route by which microbes can influence the brain is via immune system. The immune system is a critical pathway through which the gut microbiome can influence brain function and behavior. Here are some key points that illustrate this complex interaction:
- Modulation of immune responses: The gut microbiome can either activate pro-inflammatory responses or promote anti-inflammatory and immunotolerant states, depending on its composition. For instance, certain bacteria like Bacteroides fragilis can produce polysaccharide A, which promotes regulatory T cells and helps maintain immune balance.
- Neuro-immune communication: The nervous system and immune system are closely linked. Neurons can detect and respond to cytokines and other signaling molecules produced by immune cells. This bidirectional communication ensures that the brain can respond to immune challenges and vice versa.
- Behavioral responses to immune activation: Activation of the immune system can lead to sickness behaviors such as lethargy, loss of appetite, and increased sensitivity to pain, etc. These behaviors are thought to be adaptive responses that help conserve energy and promote recovery during illness. Interestingly, these responses can be triggered even in the absence of a pathogen, such as after certain vaccinations.
These interactions underscore the importance of the gut-immune-brain axis in maintaining overall health and influencing behavior.
The Intricate Role of Macrophages in the Microbiome-Gut-Brain Axis
Research on one type of immune cells, the macrophage, illustrates how the immune system can act as a go-between from the gut microbiome to brain function.
Macrophages are mobile cells associated with many organs of the body, including the gut wall and they provide protection against invasive microorganisms. Macrophages are indeed pivotal in the immune response, acting as both defenders against pathogens and regulators of inflammation. They respond to cytokines (small proteins produced by various cells types of the human body), which are signaling molecules that orchestrate the immune response. Pro-inflammatory cytokines can be induced by certain gut bacteria, leading to an inflammatory state.
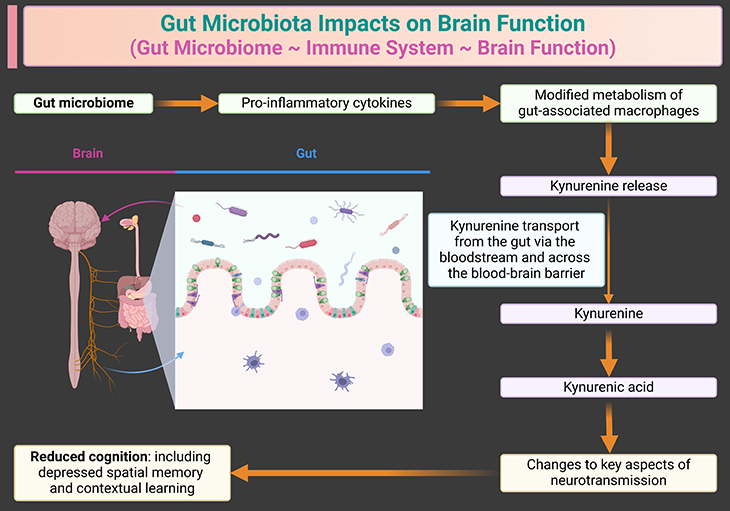
Figure 5. Modulation of gut immune cells by the gut microbiome can influence cognition in the brain. The gut microbiome plays a crucial role in modulating immune responses, which in turn can affect brain function and cognition. The interaction between gut microbiota and immune cells like macrophages is a key part of this process. When the gut microbiome is in a pro-inflammatory state, it can lead to increased production of kynurenine by macrophages. Kynurenine is a metabolite that can cross the blood-brain barrier and influence brain chemistry, potentially leading to cognitive impairments.
When macrophages are activated by pro-inflammatory cytokines, they produce kynurenine. The kynurenine pathway is a major route of tryptophan metabolism [4]. This molecule can cross the blood-brain barrier and is subsequently converted into neuroactive metabolites like kynurenic acid. These metabolites play a role in modulating neurotransmission and can impact cognitive functions such as learning and memory.
While moderate levels of kynurenine and its metabolites are essential for normal brain function, excessive levels can be detrimental. High concentrations of kynurenine, often resulting from chronic inflammation, can suppress key neurotransmission processes, potentially leading to cognitive impairments (see Figure 5).
Thus, the composition of the gut microbiome significantly influences the production of cytokines and, consequently, the activity of macrophages. A balanced microbiome promotes a healthy immune response, whereas dysbiosis (an imbalance in the microbiome) can lead to excessive inflammation and altered kynurenine metabolism.
These facts indicates the complex interplay between the gut microbiome, immune system, and brain function.
Role of Nutrition and Metabolism in Microbiome-Gut-Brain Axis
The gut microbiome can also affect behavior through its influence on nutrition and metabolism. One route that is mechanistically trivial but behaviorally important concerns microorganism which provide essential nutrients. Certain gut microorganisms synthesize essential nutrients that the host cannot produce on its own. When there’s a deficiency in these nutrients, either due to diet or microbial imbalance, it can trigger specific hunger and foraging behaviors. This adaptive response ensures that the host seeks out and consumes foods rich in the missing nutrients or a shift in food choice (see Figure 6).
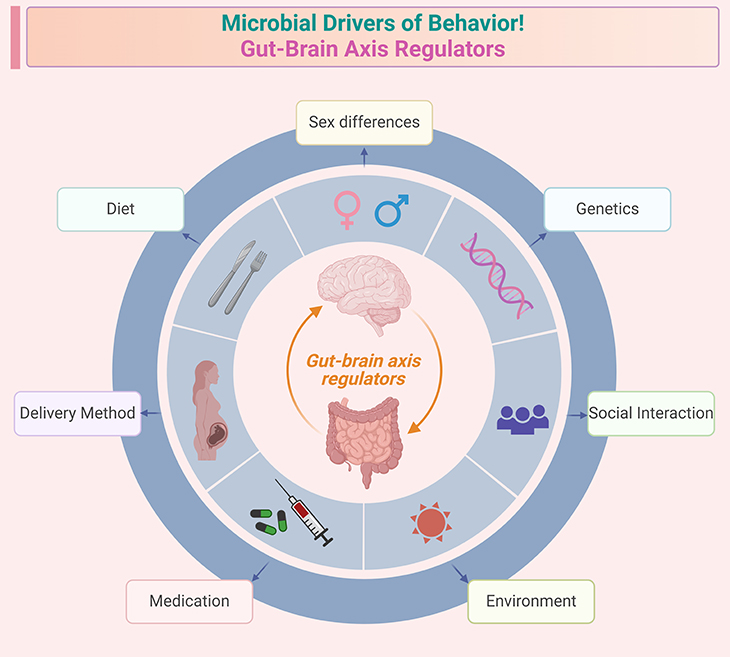
Figure 6. Microbial drivers of behavior ~ Gut-brain axis regulators. Illustration identifies common factors influencing the gut-brain axis and the behaviors affected by its perturbation. Factors influencing microbiota-gut-brain activity: (i) diet – nutrient intake, fiber, probiotics, and overall dietary patterns; (ii) congenital heredity and epigenetics – genetic predisposition and epigenetic modifications; (iii) environment – exposure to pollutants, toxins, and overall living conditions; (iv) medications – antibiotics, SSRIs, and other pharmaceuticals; (v) exercise – physical activity levels and types of exercise; (vi) mode of delivery at birth – vaginal birth versus cesarean section. Behaviors affected by microbiota-gut-brain axis perturbation: (i) cognitive behaviors – learning, memory, and decision-making; (ii) social behaviors – sociability, communication, and interaction; (iii) stress – response to stressors and overall stress levels; (iv) fear – anxiety, fear responses, and phobias; (v) food intake – appetite, eating habits, and food preferences.
The gut microbiome can affect host metabolism, including blood sugar regulation. In Drosophila flies, for instance, the presence and composition of gut bacteria have been shown to influence locomotion by modulating blood sugar levels. These changes in blood sugar can affect the activity of specific neurons in the brain that control movement [5].
Gut bacteria produce various metabolites, such as short-chain fatty acids (SCFAs), which can influence brain function and behavior. These metabolites can cross the blood-brain barrier and affect neurotransmitter systems, thereby impacting mood, cognition, and overall behavior. The diet significantly shapes the composition and function of the gut microbiome (see Figure 6). For example, diets high in fiber promote the growth of beneficial bacteria that produce SCFAs, which have anti-inflammatory and neuroprotective effects.
These facts underscore the complex and dynamic relationship between the gut microbiome, nutrition, metabolism, and behavior.
The Role of Maternal Microbiota in Fetal and Offspring Neurodevelopment
Finally, There is a very special route by which the gut microbiome can influence the brain development of the fetus, particularly through the placenta during pregnancy, ultimately, the behavior of the offspring after birth (see Figure 6) [6].
Studies on germ-free mice have shown that the absence of gut microbiota can lead to altered neural circuits, especially in the thalamus, which is crucial for relaying sensory information to the brain cortex, where many behavior decisions are made. These abnormal connections are laid down to the developing fetus of germ-free mothers; after the offspring are born, they display lifelong problems in sensing touch, even if they are provided with gut microbes.
The introduction of specific bacteria, such as clostridia, to the mother can mitigate these effects. Clostridia release small molecules that cross the placenta and influence fetal brain development. This interaction highlights the profound impact that material microbiota can have on the offspring’s neural development and subsequent behavior. (Cf. previous blogs entitled as: “Developmental Origins of Health and Disease: Neonatal Gut Microbiome ~ Day 0 to 30” & “Developmental Origins of Health and Disease: Infant Gut Microbiome ~ Day 31 to 364” & “Developmental Origins of Health and Disease: Transition from Infant to Child Microbiome ~ Day 365 to 1,000 Beyond”).
Researchers are actively investigating how these microbial molecules affect neuronal growth and connectivity in the fetal brain. This line of inquiry could lead to new insights into neurodevelopmental disorders and potential therapeutic interventions [7].
In the upcoming blog, we will address how the combined study of humans and laboratory animals has in fact provided compelling evidence that disruptions in the gut microbiome, know as dysbiosis, can contribute to various neurodevelopmental disorders, particularly autism spectrum disorder (ASD) and neurodegenerative diseases, including Alzheimer’s and Parkinson’s diseases, as well as mental health conditions, such as depression and anxiety disorders [8-9]. The potential for microbial therapy, such as probiotics or fecal microbiota transplantation, offers a promising avenue for treating these conditions by restoring a healthy gut microbiome balance.
Take Home Messages
Bidirectional communication
- The gut and brain communicate through neural, immune, and endocrine pathways, with the vagus nerve playing a crucial role in this interaction.
Influence on behavior
- The gut microbiome can affect behavior by modulating neurotransmitter production, immune responses, and metabolic processes.
Critical developmental windows
- Early childhood is a critical period when the gut microbiome significantly influences neurodevelopment. Disruptions during this time can have long-term effects on brain function and behavior.
Neurodevelopmental and neurodegenerative disorders
- Alterations in the gut microbiome are linked to various neurological disorders, including autism spectrum disorder (ASD) and Alzheimer’s disease.
Potential interventions
- Probiotics, prebiotics, and dietary modifications are being explored as potential strategies to support healthy brain development and mitigate neurological disorders.
These points highlight the complex and dynamic relationship between the gut microbiome and brain function, emphasizing the importance of maintaining a healthy gut microbiome for overall well-being.
Summary and Conclusions
In this blog we have briefly highlighted the current understanding and challenges of the microbiome-gut-brain axis.
First, critical role in brain development and function – the gut microbiota is essential for the proper development and maintenance of brain function. Disruptions in the microbiome can impact neurodevelopment and contribute to various neuropsychiatric, neurodevelopmental, and neurodegenerative diseases.
Second, early stage of research – while there is accumulating evidence from both animal and clinical studies, it is still early days in this field. Caution is needed in interpreting these studies, as the exact mechanisms and causative relationships are not fully understood.
Third, clinical applications – currently, irritable bowel syndrome (IBS) is the only condition where targeting the microbiota has shown clinical improvement in placebo-controlled trials. More research is needed to determine the optimal dosing, strain, and timing for psychobiotics and other microbiota-targeted therapies.
Next, need for rigorous studies – the field needs to move beyond correlative analyses to prospective longitudinal studies, causative and mechanistic analyses, and larger-scale trials to validate potential therapeutic approaches.
Then, defining healthy microbiota – one of the major challenges is defining what constitutes a healthy microbiota, given the significant inter-individual differences. This variability also presents opportunities for personalized medicine approaches.
Finally, diet-microbiota-gut-brain axis – diet plays a crucial role in modulating the microbiota, which in turn affects brain health. This highlights the potential for dietary interventions to support mental health, encapsulated in the idea that “let food for your microbes be thy brain medicine!”
For information on autism monitoring, screening and testing please read our blog.
References
- Dinan TG, Cryan JF. Mood by microbe: towards clinical translation. Genome Med. 2016 Apr 6;8(1):36. doi: 10.1186/s13073-016-0292-1. PMID: 27048547; PMCID: PMC4822287.
https://pubmed.ncbi.nlm.nih.gov/27048547/ - Cryan JF, O’Riordan KJ, Cowan CSM, Sandhu KV, Bastiaanssen TFS, Boehme M, Codagnone MG, Cussotto S, Fulling C, Golubeva AV, Guzzetta KE, Jaggar M, Long-Smith CM, Lyte JM, Martin JA, Molinero-Perez A, Moloney G, Morelli E, Morillas E, O’Connor R, Cruz-Pereira JS, Peterson VL, Rea K, Ritz NL, Sherwin E, Spichak S, Teichman EM, van de Wouw M, Ventura-Silva AP, Wallace-Fitzsimons SE, Hyland N, Clarke G, Dinan TG. The Microbiota-Gut-Brain Axis. Physiol Rev. 2019 Oct 1;99(4):1877-2013. doi: 10.1152/physrev.00018.2018. PMID: 31460832.
https://pubmed.ncbi.nlm.nih.gov/31460832/ - Everett BA, Tran P, Prindle A. Toward manipulating serotonin signaling via the microbiota-gut-brain axis. Curr Opin Biotechnol. 2022 Dec;78:102826. doi: 10.1016/j.copbio.2022.102826. Epub 2022 Oct 28. PMID: 36332346.
https://pubmed.ncbi.nlm.nih.gov/36332346/ - Schwarcz R, Bruno JP, Muchowski PJ, Wu HQ. Kynurenines in the mammalian brain: when physiology meets pathology. Nat Rev Neurosci. 2012 Jul;13(7):465-77. doi: 10.1038/nrn3257. PMID: 22678511; PMCID: PMC3681811.
https://pubmed.ncbi.nlm.nih.gov/22678511/ - Krajmalnik-Brown R. A Fruitful Discovery: Can Gut Bacteria Control Hyperactive Behavior? Mol Cell. 2019 Feb 7;73(3):395-397. doi: 10.1016/j.molcel.2019.01.031. PMID: 30735653.
https://pubmed.ncbi.nlm.nih.gov/30735653/
- Frerichs NM, de Meij TGJ, Niemarkt HJ. Microbiome and its impact on fetal and neonatal brain development: current opinion in pediatrics. Curr Opin Clin Nutr Metab Care. 2024 May 1;27(3):297-303. doi: 10.1097/MCO.0000000000001028. Epub 2024 Mar 12. PMID: 38488112; PMCID: PMC10990016.
https://pubmed.ncbi.nlm.nih.gov/38488112/ - Wang Q, Yang Q, Liu X. The microbiota-gut-brain axis and neurodevelopmental disorders. Protein Cell. 2023 Oct 25;14(10):762-775. doi: 10.1093/procel/pwad026. PMID: 37166201; PMCID: PMC10599644.
https://pubmed.ncbi.nlm.nih.gov/37166201/
- Srikantha P, Mohajeri MH. The Possible Role of the Microbiota-Gut-Brain-Axis in Autism Spectrum Disorder. Int J Mol Sci. 2019 Apr 29;20(9):2115. doi: 10.3390/ijms20092115. PMID: 31035684; PMCID: PMC6539237.
https://pubmed.ncbi.nlm.nih.gov/31035684/
- Socała K, Doboszewska U, Szopa A, Serefko A, Włodarczyk M, Zielińska A, Poleszak E, Fichna J, Wlaź P. The role of microbiota-gut-brain axis in neuropsychiatric and neurological disorders. Pharmacol Res. 2021 Oct;172:105840. doi: 10.1016/j.phrs.2021.105840. Epub 2021 Aug 24. PMID: 34450312.
https://pubmed.ncbi.nlm.nih.gov/34450312/