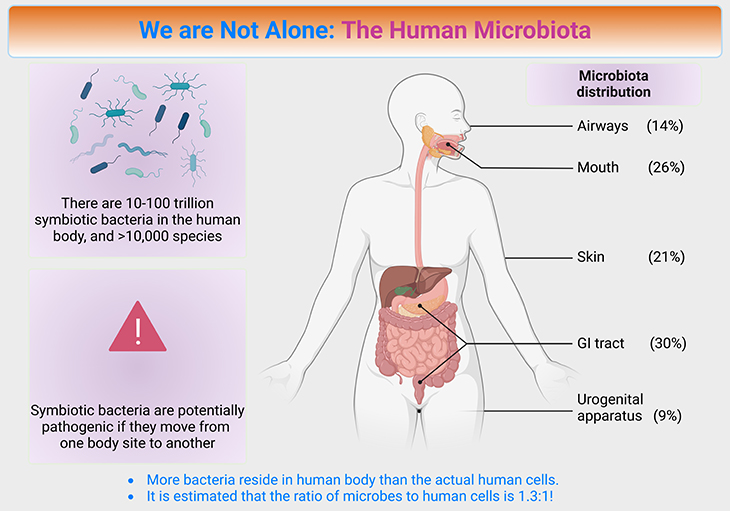
Figure 1. We are not alone ~ the human microbiota. The microbiome of many animals, including humans, are predominantly found in the gut. For example, most of the microbial partners of humans are in one region of the gut, the colon (also known as the large intestine). The skin and upper airways are also extensively colonized, as is the vagina of women, but the internal organs such as the liver and brain bear few or no microorganisms in healthy individuals. The total weight of microorganisms in an adult human is estimated at 2-6 lb (1 – 3.7 kg), which is approximately 3% of body weight; for comparison, the adult brain or liver each weigh 3 lb (1.4 kg). Half of the estimated 80 trillion cells in the human bodies are microbial and mostly bacteria. However, microorganisms do not account for half of our weight because most microbial cells are much smaller than human cells.
Introduction
In general, we like to think that we are human, but that is only partly true. Half of the estimated 80 trillion cells in the human body are microorganisms (see Figure 1). These microorganisms are crucial for our health and wellbeing. They protect us from (i) pathogens, (ii) support our metabolism, (iii) immune system, and (iv) influence our mood and emotional state. Besides, bearing microorganisms is not special to humans. All healthy animals and plants are inhabited by communities of microorganisms known as microbiomes, and usually, these microorganism contribute to good health.
Factors Affecting the Infant Gut Microbiome ~ Day 31 to 364
The initial seeding of the microbiome in the prenatal stages and through birth represents an important period in the colonization of the newborn infant. However at this stage the deliberate manipulation of the gut microbiome is generally not feasible, with little scope for clinical interventions (cf. previous blog entitled as: ‘Developmental Origins of Health and Disease: Neonatal Gut Microbiome ~ Day 0 to 30.’).
Simply put, while a range of variables will affect the initial exposure to microorganisms, such variables are often difficult to control, for example, birth mode and gestation. While the longer-term influence of such variables is important to understand. From a medical perspective, it is of paramount importance to comprehensively understand how a single or combination of interventions will influence the developing gut microbiome in the neonate and infant stages of life [1].
Following birth and over the first months of life, the gut microbiome is chaotic and highly dynamic, with large shifts in the overall bacterial community potentially occurring from day to day. This early stage of life represents a key window for the manipulation and long-term establishment of a stable and diverse community, which is typically regarded as stable from childhood and throughout adulthood.
The gold standard for establishing a healthy gut microbiome is thought to result from full term, vaginally delivered, and breastfed (FTVDBF) infants. During the infant stage, FTVDBF infants tend to have relatively high abundance of the following microbes, for instance:
- Bifidobacterium,
- Bacteroides,
- Clostridium, and
- Atopobium.
The percentage of facultative to strict anaerobes in the FTVDBF infant gut microbiome at day 90 is 60% to 40%, respectively.
Here we portray this key phase of life, focusing on variables that can generally be controlled, such as feeding, antibiotics, and supplementation. As well as to understand the mechanisms of these interventions – for understanding the role of the gut microbiome in programming long-term host health.
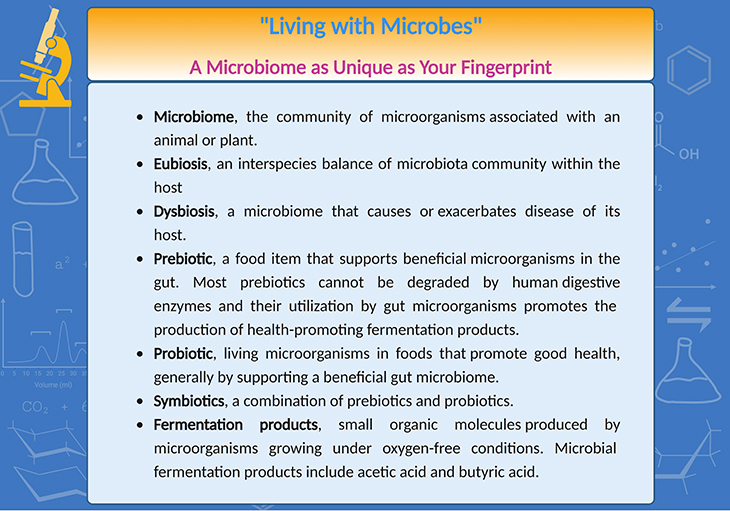
Box-1: A microbiome as unique as your fingerprint.
(1) Infant Feeding Mode
Human milk-oriented gut microbiota
Given the importance of seeding the microbiome with beneficial bacteria, it is not surprising that breast milk contains a range of beneficial microorganisms, including Lactobacilli and Bifidobacteria. Historically assumed to be sterile, more than 700 different bacterial species have been detected in human milk, commonly including species from the following genera, viz:
- Weissella,
- Leuconostoc,
- Staphylococcus,
- Streptococcus, and
- Lactococcus.
The importance of feeding regime for the early development of the gut microbiome became apparent when researchers realized that the bacteria of the stool did not resemble the mode of delivery after the initial week of life. As discussed in our previous blog (cf. previous blog entitled as: ‘Developmental Origins of Health and Disease: Neonatal Gut Microbiome ~ Day 0 to 30.’), Lactobacillus represents a major genus of the vaginal microbiome, but this genus is relatively low in abundance in the gut microbiome. Furthermore, it has been shown that specific bacterial strains transferred from maternal breast milk successfully colonize the neonatal gut.
While relatively conserved compared to other sample types, for example, stool, the microbial community is breast milk is different between different mothers and can change over time within the same individuals. It has been determined that most abundant genera of bacteria in human milk from American mothers were, for example:
- Streptococcus,
- Staphylococcus,
- Serratia, and
- Corynebacteria,
and notably lower levels of Lactobacilli and Bifidobacteria compared to European cohorts. Even within the same geographical location, differences in the number and diversity of bacteria in the breast milk microbiome arising from dietary influences have been reported in both humans and animals.
In addition to the direct passage of viable microbes, human milk also confers protection against pathogens through the transmission of maternal immunoglobulin A (IgA). IgA is the first source of antibody-mediated immune protection in the neonatal gut and has been shown to prevent the translocation of bacteria as well as promote long-term gut homeostasis by regulating the gut microbiome (see Figure 2) [2].
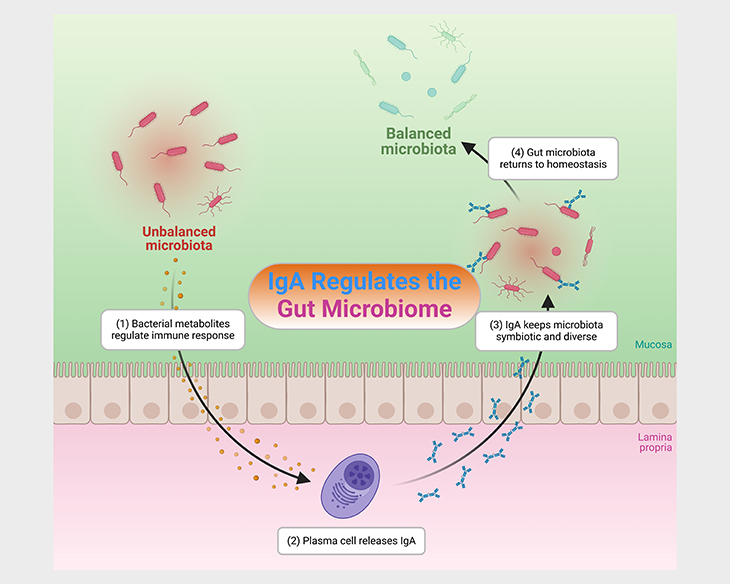
Figure 2. IgA – microbiota homeostasis. Immunoglobulin A (IgA) mediates microbial homeostasis at the intestinal mucosa. Within the gut, IgA acts in a context-dependent manner to both prevent and promote bacterial colonization and to influence bacterial gene expression, thus providing exquisite control of the microbiota. IgA-microbiota interactions are highly diverse across individuals and populations, yet the factors driving this variation remain poorly understood. Disrupted secretory IgA – microbiota interactions in the gut have been linked to: (i) elevated risk of allergies and asthma in children, (ii) increased inflammatory bowel disease (IBD) severity, (iii) higher infectious risk, and most recently, (iv) altered responses to malnutrition.
Human milk oligosaccharides contribute to shaping microbial communities
The distinctive composition of the gut microbiome in breastfed babies can be attributed to the remarkable composition of the sugars in human milk. The dominant sugar in the milk of all mammals is lactose, which is made up of two sugar units, viz., glucose and lactose. Lactose is easily digested by baby mammals; and is a major source of energy for growth (see Figure 3; Table 1; and Box – 1).
Milk also contains small amounts of other sugars, including oligosaccharides, which comprise three to six sugar units. The oligosaccharides content of human milk is very high (5-20 g per liter), and includes three additional components, (viz., fucose, acetylglucosamine and a sialic acid) which, along with glucose and galactose, are linked together in many different ways. More than 100 different kinds of human milk oligosaccharides (HMOs) have been described.
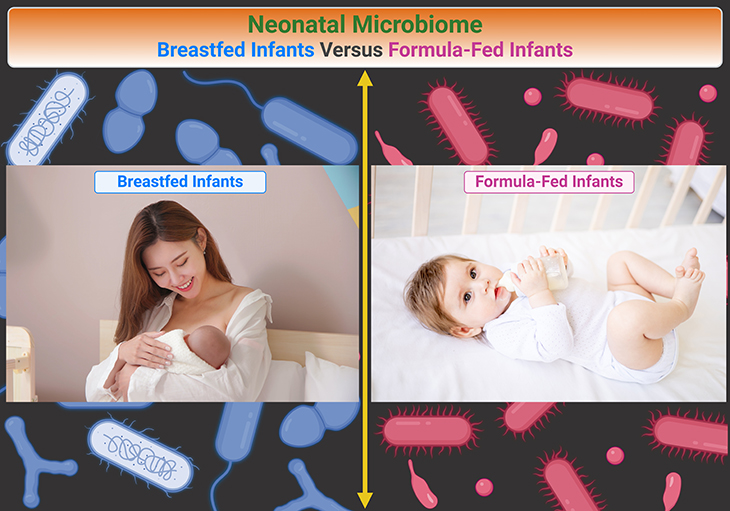
Figure 3. Human milk and the gut microbiome. Comparison of breast milk and formula on the neonatal microbiome. The gut microbiome differs between breastfed babies and babies fed on formula milk (which is derived from cow’s milk). There are much higher levels of certain bacteria, especially Bifidobacterium species, and lower overall diversity of bacteria in the gut microbiome of breastfed babies. It is widely recognized that certain Bifidobacterium species support infant health by protecting against pathogens and regulating immune functions, and this most likely contributes tothe low incidence of allergic diseases in children that were breastfed as babies.
HMOs are important because, unlike lactose, the baby cannot digest HMOs. They are passed without modification into the colon, where they are utilized by bacteria, especially Bifidobacterium. The different HMOs support the growth of different Bifidobacterium species and strains, and some HMOs can only be broken down by several species working together.
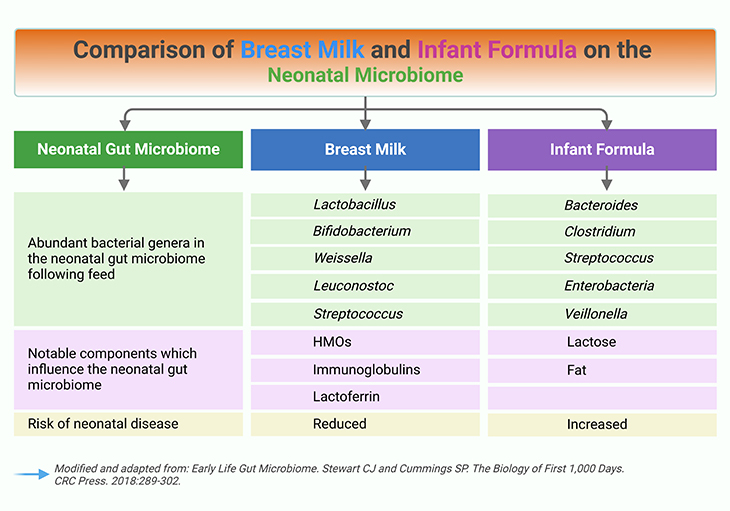
Table 1. Comparison of breast milk and formula on the neonatal microbiome.
Because of these HMOs, human milk is a natural prebiotic food, meaning that it feeds beneficial microorganisms in the gut. Interestingly, the HMO composition of human milk varies among different mothers, and this may contribute to the variation in the gut microbiome composition among infants. There is no evidence that the differences in HMO profiles in the milk of different mothers influence the health of their babies [3].
As well as promoting beneficial bacteria, HMOs can suppress some harmful bacteria. For example, they inhibit the growth of group B Streptococcus, which can cause sepsis and meningitis in newborn babies. HMOs also prevent the attachment of diarrhea-causing bacteria, such as Campylobacter, to the gut wall of babies, thereby protecting infants against diarrheal illnesses (see Figure 3; Table 1; and Box – 1).
The high levels of and complexity of oligosaccharides of human milk is most unusual, compared to the milk of other mammals. The milk of some other primates is enriched in oligosaccharides, but less so than humans. The oligosaccharides content of cow’s milk is 100-1,000 times lower than in human milk, and this is a major factor contributing to the low abundance of Bifidobacterium in babies fed on formula milk.
(2) Disease and Antibiotics
The number of infants death in the United States is around 6 infants for every 1,000 births. A major cause of infant death is prematurity, and the increasing number of infants surviving preterm birth has presented new problems.
Despite increased and novel research efforts, little improvement has been made in the rate of mortality due to preterm disease such as necrotizing enterocolitis (NEC) and sepsis, which accounts for >20% of all preterm mortality. Significant morbidity associated with prematurity, such as delayed growth and cognitive and neurological function, are also common to infants delivered preterm.
To reduce the risk of infections, vulnerable neonates may undergo a short course of antibiotics. Concerns regarding antibiotic treatment related to the gut microbiota include (a) the spread of antibiotic resistance among pathogens, and (b) that alteration of the microbiota will interfere with human-microbe interactions that are fundamental to human development.
Antibiotics exposure and risk for later life disease
Perturbation of the microbiota by antibiotic exposure has long-lasting and potentially irreversible effects on microbiome community development, and increases the risk of neonatal disease [4], such as:
- obesity,
- asthma,
- type I diabetes, and
- other immune-mediated diseases.
The relationship between antibiotic perturbation of the microbiome and alterations to host immune system function have been described. For instance, in humans,intrapartum antibiotic exposure can result in a depletion of Bacteroidetes, offset by an enrichment of Firmicutes, which may persist to 1 year of age depending on the antibiotic class.
Additionally, infant antibiotic use increases risk of (i) pathobiont colonization and (ii) delayed microbiome maturation. This may be a causal factor in the development of Crohn’s disease and other dysbiosis-associated diseases due to lower levels of Clostridiales and Ruminococcus, both of which are prominent short-chain fatty acid (SCFA) producers (see Figure 4).
SCFAs, such as acetate, propionate, and butyrate, are bacterial products of complex carbohydrate metabolism. SCFAs can be utilized by the host, for example:
- as a source of energy,
- play a role in the regulation of gene expression,
- are anti-inflammatory, and
- may influence lipid metabolism, and
- the immune system.
(3) Supplementation
As appreciation for the significance of the gut microbiome in health and disease has risen in recent years, so too has the interest in manipulating its development with probioticsand prebiotics(see Figure 4; and Figure 5).
Probiotics are live microorganisms, which can colonize the host and provide benefits to health, both directly, for example, producing a substrate for the host; and indirectly, for instance, preventing the overgrowth of potential pathogens. Prebiotics are substrates that promote the growth of beneficial bacteria. Symbiotics are a combination of prebiotics and probiotics (see Box -1) [5-7].
The neonatal and infant stages represent the most important windows for establishing beneficial microbes in the gastrointestinal tract. It has been suggested that in the next decade the use of probiotics in early life will be routine practice in the most vulnerable populations. The use of probiotics and prebiotics in preterm infants seems particularly important for establishing long-term health, although recent large trials have produced opposing results.
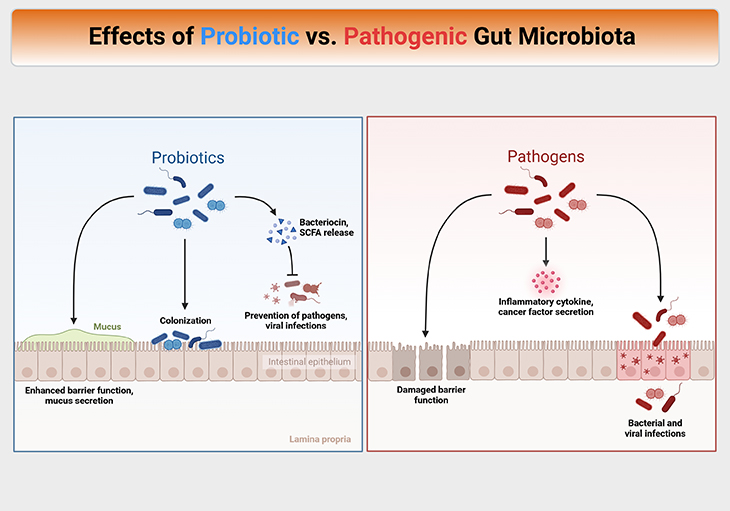
Figure 4. Effects of probiotic versus pathogenic gut microbiota. Mechanisms of action of probiotics and prebiotics are complex, diverse, heterogeneous, and often strain- and compound-specific. Probiotics interact with both the host and microbiome via molecular effectors present on the cell structure or secreted as metabolic products. Probiotic metabolites can act on the microbiota (i) by cross feeding interactions, (ii) changes in the gastrointestinal microenvironment (e.g., pH lowering), (iii) competition for nutrients and binding sites, and (iv) inhibition of growth via the production of strain-specific antibacterial compounds including bacteriocins. Such microbiota-directed effects contribute to the ability of probiotics to mediate health benefits in pathogen overgrowth states such as vaginal and oral dysbioses.
While results are still emerging, it has been shown that probiotics can profoundly shift the gut microbiome in neonates, unsurprisingly due to much larger relative abundances of the probiotic species in the probiotic recipients. The evenness of the community also tends to increase, indicating increased ecological stability.
It should be noted that the probiotics have been trialed for the prevention of allergic disease in mothers and infants, with an apparent reduced incidence of eczema, but not asthma, allergy, or other allergic conditions. Overall, probiotics are really shown to have negative consequences and, at worst, tend to have no overall effect.
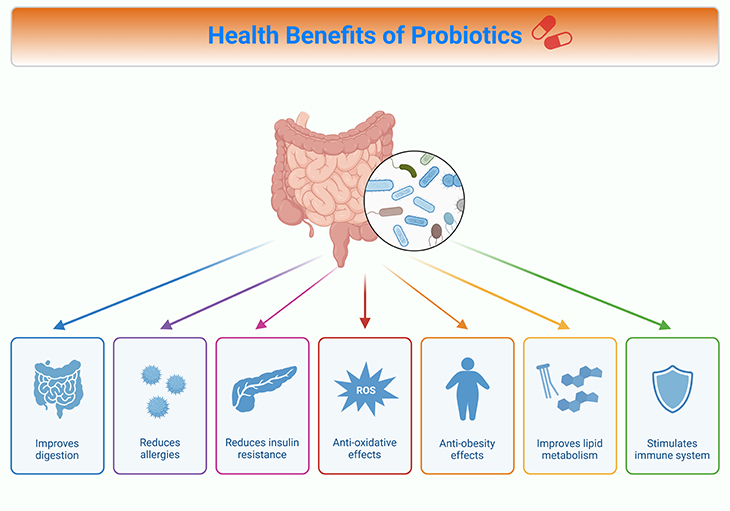
Figure 5. Health benefits of probiotics. Proposed mechanisms of probiotic action include: (a) enhanced epithelial barrier function, (b) enhanced mucosal IgA response, (c) direct antagonism against pathogens, (d) competitive exclusion of pathogens, (e) prevention of apoptosis, (f) production of anti-inflammatory cytokines, and (g) downregulation of proinflammatory pathways. However, the exact mechanism of probiotics is probably strain dependent.
Although less researched than probiotics, the potential for prebiotic to modulate a healthy gut microbiome is huge. As discussed above, much of the beneficial nature of breast milk is thought to result from its prebiotic properties. However, supplementation of HMOs to the feed of preterm infants did not result in the expected increase in bifidobacteria [1; 5-6].
For infancy, one of the most prominent probiotics is lactoferrin, which is known to help protect against infection and promote nutritional status. Lactoferrin has been shown to specifically inhibit pathogenic bacteria in the gut microbiome by scavenging free iron to such an extent that remaining concentrations are too low to enable the growth of pathogenic Escherichia Coli. The effects of prebiotics on the overall gut microbiome structure will emerge in the coming years.
Take Home Messages
We are not alone ~ Living with microbes
- The gut microbiome of humans and animals, predominantly found in the colon, plays a crucial role in health and wellbeing by protecting against pathogens, supporting metabolism, influencing the immune system, and affecting mood and emotional state.
Human milk ~ Gut microbiome
- Breastfeeding is essential for seeding the infant gut microbiome with beneficial bacteria, such as Bifidobacterium and Lactobacillus, which contribute to a stable and diverse microbial community.
- Human milk contains oligosaccharides that act as prebiotics, promoting the growth of beneficial bacteria and protecting against pathogens.
Antibiotics exposure ~ Later life disease
- Antibiotic exposure in infancy can have long-lasting effects on the gut microbiome, increasing the risk of diseases like obesity, asthma, and immune-mediated conditions.
- Antibiotics can alter the microbiota composition, leading to pathobiont colonization and delayed microbiome maturation.
Nutraceutical products ~ Probiotic/Prebiotic supplementation
- Probiotics, live microorganisms that provide health benefits, and prebiotics, substrates that promote the growth of beneficial bacteria, are being explored to manipulate the gut microbiome for improved health outcomes in infants.
- Probiotics can shift the gut microbiome composition, while prebiotics like human milk oligosaccharides have potential prebiotic properties.
Infant gut microbiome ~ Main drivers of the microbial colonization
- The establishment of the infant gut microbiota is influenced by factors like maternal inheritance of specific microorganisms, dietary compounds in human milk, mode of delivery, host genetics, gestational age, and maternal diet.
- Understanding these factors and interventions like probiotics and prebiotics can play a crucial role in shaping long-term host health through the gut microbiome.
Summary and Conclusions
The gut microbiota represents a prime example of how an environment which is considered sterile, or at least poorly colonized, at birth is rapidly occupied by a plethora of microbial communities. Notably, this colonization appears to follow typical trajectories that are governed by stochastic processes and microbe-host coevolution forces.
In this context, only specific microorganisms that are maternally inherited are, under natural circumstances, driving the establishment of the infant gut microbiota. The subsequent development of this early gut microbiota is then modulated by specific dietary compounds present in human milk that support selective colonization.
It has been shown that the genomes of infant gut commensals, in particular bifidobacteria, are genetically adapted to utilize specific glycan components of this human secretory fluid, the milk. This represents a very intriguing example of host-microbe coevolution, where both partners are believed to benefit.
There is growing evidence that such a mechanism, by which host products act as key agents for the modulation of the gut microbiota, thus acting as natural prebiotics, is a common scheme not limited to humans or other primates but extending to all species across the mammalian tree of life.
Notably, other maternally linked forces responsible for the establishment of the very early infant gut microbiota include the mode of delivery, host genetics, gestational age, and maternal diet.
There are intriguing indications that the infant gut is not always sterile at delivery and that fetal colonization might sometimes occur, with a concurrent transfer of maternal microbiota to the fetus during pregnancy.
In this context, the early stages of life represent a more opportunistic period of human life where the gut microbiota may be more prone to changes by interventions involving probiotics, prebiotics, phages, or combinations of these.
For information on autism monitoring, screening and testing please read our blog.
References
- Milani C, Duranti S, Bottacini F, Casey E, Turroni F, Mahony J, Belzer C, Delgado Palacio S, Arboleya Montes S, Mancabelli L, Lugli GA, Rodriguez JM, Bode L, de Vos W, Gueimonde M, Margolles A, van Sinderen D, Ventura M. The First Microbial Colonizers of the Human Gut: Composition, Activities, and Health Implications of the Infant Gut Microbiota. Microbiol Mol Biol Rev. 2017 Nov 8;81(4):e00036-17. doi: 10.1128/MMBR.00036-17. PMID: 29118049; PMCID: PMC5706746.
https://pubmed.ncbi.nlm.nih.gov/29118049/ - Huus KE, Petersen C, Finlay BB. Diversity and dynamism of IgA-microbiota interactions. Nat Rev Immunol. 2021 Aug;21(8):514-525. doi: 10.1038/s41577-021-00506-1. Epub 2021 Feb 10. PMID: 33568782.
https://pubmed.ncbi.nlm.nih.gov/33568782/ - Dombrowska-Pali A, Wiktorczyk-Kapischke N, Chrustek A, Olszewska-Słonina D, Gospodarek-Komkowska E, Socha MW. Human Milk Microbiome-A Review of Scientific Reports. Nutrients. 2024 May 8;16(10):1420. doi: 10.3390/nu16101420. PMID: 38794658; PMCID: PMC11124344.
https://pubmed.ncbi.nlm.nih.gov/38794658/ - Tanaka M, Nakayama J. Development of the gut microbiota in infancy and its impact on health in later life. Allergol Int. 2017 Oct;66(4):515-522. doi: 10.1016/j.alit.2017.07.010. Epub 2017 Aug 18. PMID: 28826938.
https://pubmed.ncbi.nlm.nih.gov/28826938/ - Dominguez-Bello MG, Godoy-Vitorino F, Knight R, Blaser MJ. Role of the microbiome in human development. Gut. 2019 Jun;68(6):1108-1114. doi: 10.1136/gutjnl-2018-317503. Epub 2019 Jan 22. PMID: 30670574; PMCID: PMC6580755.
https://pubmed.ncbi.nlm.nih.gov/30670574/ - Cunningham M, Azcarate-Peril MA, Barnard A, Benoit V, Grimaldi R, Guyonnet D, Holscher HD, Hunter K, Manurung S, Obis D, Petrova MI, Steinert RE, Swanson KS, van Sinderen D, Vulevic J, Gibson GR. Shaping the Future of Probiotics and Prebiotics. Trends Microbiol. 2021 Aug;29(8):667-685. doi: 10.1016/j.tim.2021.01.003. Epub 2021 Feb 4. PMID: 33551269.
https://pubmed.ncbi.nlm.nih.gov/33551269/ - Iebba V, Totino V, Gagliardi A, Santangelo F, Cacciotti F, Trancassini M, Mancini C, Cicerone C, Corazziari E, Pantanella F, Schippa S. Eubiosis and dysbiosis: the two sides of the microbiota. New Microbiol. 2016 Jan;39(1):1-12. PMID: 26922981.
https://pubmed.ncbi.nlm.nih.gov/26922981/