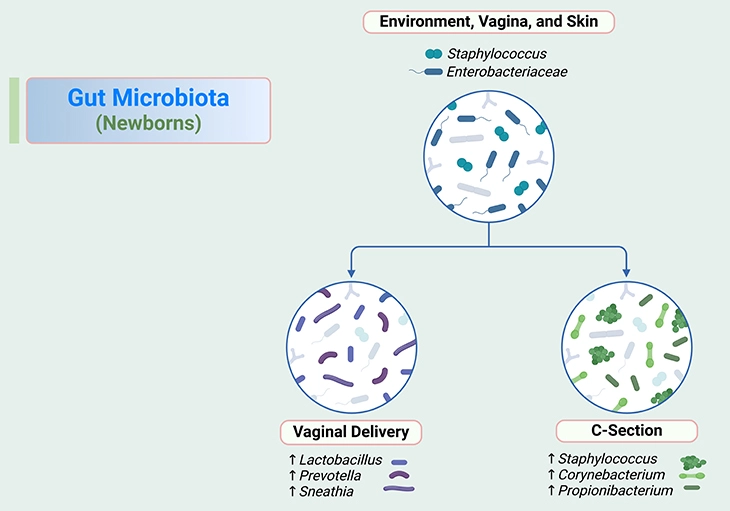
Figure 1. Gut microbiota in newborns. Gut microbiota ecology and function are dynamic in infancy but are stabilized in childhood. The ‘new friends’ have great impact on the development of the digestive tract and host immune system. In the first year of life, especially, the gut microbiota dramatically changes through interactions with the developing immune system in the gut. The process of establishing the gut microbiota is affected by various environmental factors, with the potential to be a main determinant of life-long health. Consequently, understanding the factors influencing infant gut microbiome maturation, and how these changes impact later health, is an important first step towards preventing transgenerational risk that underlies insulin resistance, diabetes, obesity, non-alcoholic fatty liver disease, as well as diseases associated with autoimmunity in youth, including type I diabetes, asthma and allergies – in children.
Introduction
The human body has trillions of microbes inhabiting every surface exposed to the environment, both inside and out, termed the microbiome (micro, small; biom, community in a distinct environment). The largest of the community of microbes is found within the gastrointestinal tract where the gut microbiome in adults is estimated to harbor 1014 bacterial cells, which roughly equates to 100 to 300 times the number of genes in the human genome. This collection of microbes assembles largely through chance, but the assembly is not without design.
Microbes have existed on earth for 3.5 billion years, based on the oldest discovered fossilized evidence; humanity has therefore coevolved with its microbial flora, and they play a pivotal role in the neonate, facilitating (i) maturation of the immune system, (ii) protection from potential pathogens, and (iii) digestion of gut contents for the synthesis of essential vitamins and nutrients (see Figure 1).
Large-scale studies of human populations, such as the Human Microbiome Project (HMP), have revealed that the human microbiome is unique to an individual and remains highly stable through adulthood in healthy individuals. Research is now focused on how perturbations in normal microbiome development occur and the subsequent risk factors associated with a range of diseases.
The initial development of the microbiome has important consequences for the immediate and long-term health. The infant gut microbiome is thought to reach adult-like profiles at around 3 years of age. Thus, the first 1,000 days of life are critical, and this blog addresses the factors influencing gut microbiome development through this time (see Figure 2).
Factors Affecting the Neonatal Gut Microbiome ~ Day 0 to 30
Under normal circumstances neonates are generally thought to be sterile in the intrauterine environment, with the first exposure to microbes occurring during birth. However, intriguing emerging evidence also suggests that bacterial DNA might be present in the placenta. Although the question remains elusive of when exactly the first exposure of the neonate to microbes takes place, the diversity of the human microbiome immediately after birth is low and increases from this point on.
The first microbes to interact with the neonate have significant roles in educating the immune system, programming healthy development, and metabolic programming. Primary colonizers of the gut microbiome include mixture of cutaneous and enteric facultative anaerobes, dominated by lactic acid bacteria including (see Figure 1 and 2), for example:
- Lactobacillus,
- Enterococcus,
- Streptococcus, and
- Staphylococci (coagulase-negative).
In a matter of days, the primary colonizing bacteria deplete the oxygen supplies within the gut, resulting in the growth of strictly anaerobic bacteria including (see Figure 1 and 2), for instance:
- Bifidobacterium,
- Clostridium, and
- Bacteroides.
During the first month of the life the development of microbiome is highly chaotic and largely stochastic. The main factors contributing to initial microbial exposure are related to the maternal microbiome, with mode of delivery and gestation also playing crucial roles. This series of blogs explores the prenatal and postnatal phases, discussing the importance of these influences on the developing neonatal microbiome.
Maternal Microbiome
There are many factors that influence the maternal microbiome at various body sites, which in turn influence the neonatal microbiome. The main factors shaping the maternal microbiome include:
- diet,
- environment (e.g., socioeconomic status)
- antibiotic exposure
For example, diet is known to have significant effects on the gut microbiome, due to altering favorable growth conditions from undigested dietary components, and likely has some influence on the oral community. Given the placental microbiome seems linked to the oral community, such effects may have direct implications to the developing fetus (see Figure 3).
The maternal gut, vaginal, and skin microbiomes all contribute to the initial exposure of viable organisms to the neonate. Again, the environment of the mother will influence the bacterial communities in each of these body sites, with variation between different geographies, socioeconomic classes, and cultural traditions having the most pronounced effects.
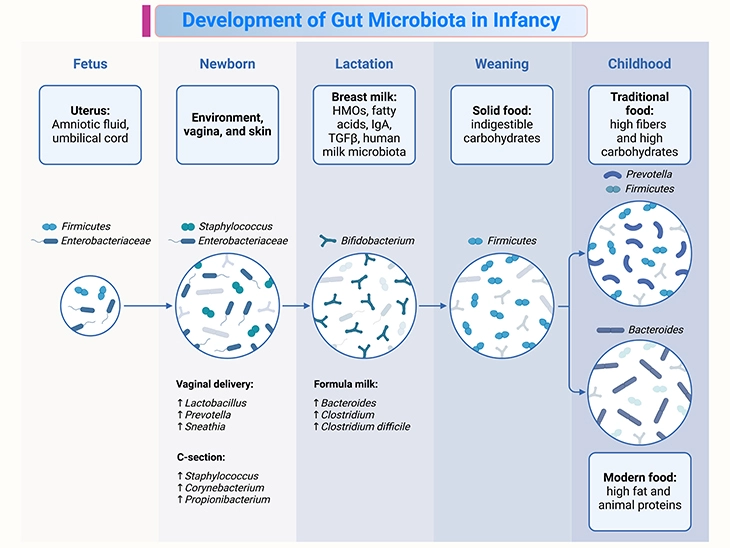
Figure 2. Development of gut microbiota in infancy. Fetal-to-childhood gut microbiota colonization and important factors affecting this process. Establishment of the gut microbiota may begin in utero and be affected by dynamic shifts in early life. Diversity of the gut microbiota increases with age until it becomes a stable adult microbiota. This process of establishing the gut microbiota is affected by various factors such as: (i) delivery mode, (ii) methods of milk feeding, (iii) the introduction of solid foods, and (iv) foods consumed daily in childhood.
Independent of the birth mode, maternal skin microbiome is transferred to the neonate through the initial stages of life from holding, cuddling, kissing, and breastfeeding. Importantly, the skin microbiome varies drastically based on the specific site, with sebaceous sites dominated by Propionibacterium and moist areas dominated by Staphylococcus and Corynebacterium.
The role of feeding in modulating the developing offspring microbiome, for example, the maternal milk microbiome is influenced by several factors, including:
- maternal weight,
- diet, and
- antibiotics.
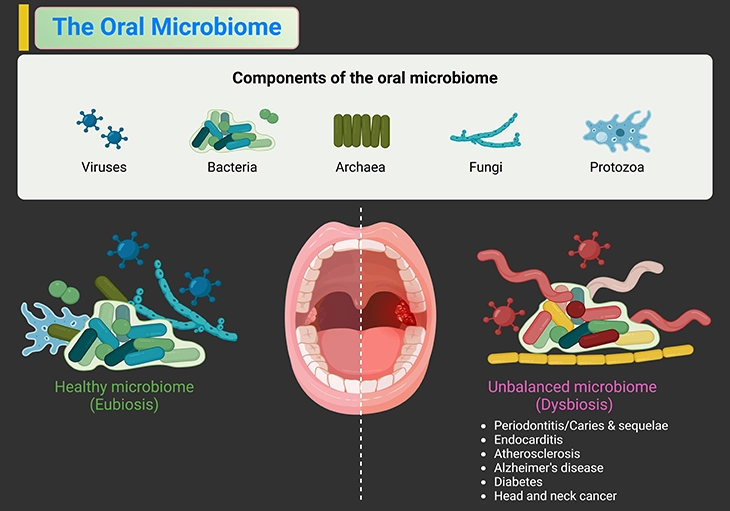
Figure 3. Acquisition and establishment of the oral microbiota. Besides bacteria, other microbes, such as fungi, archaea, viruses, the newly classified candidate phyla radiation group of ultra-small bacteria and even protozoa, are part of the microbiome. There is an increasing body of evidence that this ‘dark matter’ of the human microbiome has important functions and contributes to shaping and maintaining the microbiome and the immune system of the host. The oral microbial ecosystem is shaped in utero, by, most likely, exposure of maternal antigens to the immune system of the infant, in this way preparing the infant for postnatal microbial encounters. This makes healthy oral ecosystem of the mother of paramount importance. The modes of birth and feeding, as well as perinatal exposure to medications, determine which microbes will be encountered first and if at all. Teeth eruption and oral hygiene habits, together with sugar consumption, exposure to antibiotics, maternal smoking, and oral health status of the caregiver affect the developmental trajectories of infants’ and children’s oral microbiota. Besides these environmental factors, there is growing body of evidence that the health-associated oral microbiota has a heritable component.
Thus, even after birth, the maternal diet continues to be a significant driver of the early infant microbiome, with reduced diversity in obese and high-fat-consuming mothers. It is also well understood that the milk microbiome is not stable and changes over the course of lactation, with evidence suggesting a correlation between the early milk microbiome with the oral microbiome (see Figure 2 and 3).
In light of such evidence and association of the placental microbiome with oral bacterial communities, it is important to determine the true extent of oral communities in seeding other sites, and how therapeutic manipulation can be tailored to maximize oral health in the prenatal and postnatal stages.
Despite the numerous ways in which the maternal microbiome is altered, it is well established that full-term, vaginally delivered, breastfed neonates represents the “gold standard” for short- and long-term health.
Delivery Mode
In the first 24 hours of life, neonatal skin and gut microbiomes resemble the bacterial community from the mode of birth. In virginally delivered infants, gut microbiota resemble their mothers’ vaginal microbiotas, which are dominated by (see Figure 2), for instance:
- Lactobacillus,
- Prevotella,
- Sneathia, or
- Atopobium.
Whereas the microbiotas of infants born by caesarean section are most similar to skin microbiota, which is dominated by (see Figure 2), for example:
- Staphylococcus,
- Corynebacterium, and
- Propionibacterium.
Besides, some studies revealed that colonization by Bacteroides and Bifidobacterium is delayed for one month after birth, whereas Clostridium difficile was abundant at one month. Underrepresentation of Bacteroides was also observed in the microbiotas of infants born by caesarean section for three to four months after birth.
In addition, it has been shown that infants born by elective caesarean section had particularly low bacterial diversity. Caesarean birth has been associated with an increased risk for immune disorders such as:
- allergic rhinitis,
- asthma, and
- celiac disease.
The profound effects of birth mode on the neonatal gut microbiome are generally lost over time, typically within the first 30 days of life, with other factors overriding this initial microbiome composition.
Gestational Age
The preterm microbiota: The ‘gestational age’ refers to the number of weeks from the last menstrual cycle of the mother to the birth of infant. A normal pregnancy, where an infant is delivered full term, will last 38 to 42 weeks. Babies born at less than 37 weeks gestation are considered ‘preterm’. However, most research exploring preterm infants is focused on very low birth weight (VLBW; <1500 g) infants born at less than 32 weeks gestion.
Preterm neonates generally have a unique and unnatural beginning to life, where they are immediately housed in the incubators with limited environmental exposure. In comparison, term neonates do not generally have restricted environmental contact and will be exposed to a much greater number of microbes in the days immediately following birth. Given the importance of the developing neonatal microbiome, a number of studies have been performed to characterize the preterm gut microbiome and determine how this differs from that of full-term infants.
Microbial succession in the infants is profoundly influenced by gestational age. The overall acquisition of a stable and diverse gut microbiome is delayed in preterm infants, with preterm infants expected to have lower diversity in the first month of life compared to term infants. In general, it is unclear, however, whether the ‘immature’ preterm microbiota plays a causal role in reduced growth and elevated risk of infectious and inflammatory disorders, such as sepsis and necrotizing enterocolitis, or whether the preterm microbiota assembles as an adaptive response to such stressors.
Recent findings suggest that the preterm gut microbiota follows a three-phased pattern of assembly, as shown below:
- Phase 1 – beginning with a facultative anaerobic-dominated composition – (primarily Bacilli),
- Phase 2 – followed by expansion of obligate anaerobes and fermentation-based metabolism dominated by Gammaproteobacteria, and
- Phase 3 – Clostridia.
Transition between phases is strongly influenced by postmenstrual age (PMA), nutrition and medication usage, and delayed transition to Phase 3 is strongly associated with reduced weight-for-age Z-score. The observation that PMA strongly drives gut microbial transition in a nonrandom manner suggests that developmental factors within the mucosal immune system or intestinal metabolic environment may drive host-microbial homeostasis and mutualism.
Coupled to the increased prevalence of antibiotics in the preterm population, it is important to consider that limited environmental exposure is likely to be the primary reason for much of the contrast between preterm and term neonates.
Take Home Messages
The First 1000 Days
- The first 1000 days – the period from conception to 2 years of age – represents a critical window of early childhood growth and development.
- This prenatal and early postnatal period is defined by rapid maturation of metabolic, endocrine, neural, and immune pathways, which strongly influence and support child growth and development.
Microbiota ~ Microbiome
- An emerging perspective of human developmental biology includes the trillions of microbes (microbiota) and their genes (microbiome) that reside within the human body, and which assemble and stabilize during the first 2 years of life.
- The assembly of microbial communities within the gastrointestinal tract during early life plays a critical role in immune, endocrine, metabolic, and other host developmental pathways.
Prenatal ~ Postnatal Microbiome
- There is emerging knowledge of the influence of prenatal microbial communities on fetal and postnatal growth. Environmental insults during this period, such as food insecurity and infections, can disrupt this optimal microbial succession, which may contribute to lifelong and intergenerational deficits in growth and development.
Gut Microbiota-Targeted Intervention
- The first 1000 days provides a window of opportunity for modulating the microbiota through interventions such as diet, antibiotics, probiotics, prebiotics, or fecal microbiota transplantation to promote healthy growth and development.
Summary and Conclusions
Here we have briefly presented the rapidly growing knowledge of how the human microbiota regulates growth and developmental trajectories during the first 1000 days, especially, 0 to 30 days to start with.
Stable microbial communities are established through dynamic changes in infancy and are affected by various environmental factors, for example, the mode of delivery and methods of milk feeding. Simultaneously, the immune system matures through bidirectional interactions, with the gut microbiota promoting the development of the immune system but also the host immune system shaping the development of the gut microbiota. Alterations in the development of gut microbiota during infancy can have a variety of negative effects on immune health and the onset of allergies.
In general, the simultaneous assembly of the microbiota alongside endocrine, immune, and metabolic pathways reveals tight regulatory interdependence between microbiota and host underlying growth and development. Substantial evidence suggests that the trajectories of child growth and development are primed during the first 1000 days, or even earlier. Hence, this early-life period represents a critical window in which to focus mechanistic research and interventions.
For information on autism monitoring, screening and testing please read our blog.
References
- Tanaka M, Nakayama J. Development of the gut microbiota in infancy and its impact on health in later life. Allergol Int. 2017 Oct;66(4):515-522. doi: 10.1016/j.alit.2017.07.010. Epub 2017 Aug 18. PMID: 28826938.
https://pubmed.ncbi.nlm.nih.gov/28826938/ - Milani C, Duranti S, Bottacini F, Casey E, Turroni F, Mahony J, Belzer C, Delgado Palacio S, Arboleya Montes S, Mancabelli L, Lugli GA, Rodriguez JM, Bode L, de Vos W, Gueimonde M, Margolles A, van Sinderen D, Ventura M. The First Microbial Colonizers of the Human Gut: Composition, Activities, and Health Implications of the Infant Gut Microbiota. Microbiol Mol Biol Rev. 2017 Nov 8;81(4):e00036-17. doi: 10.1128/MMBR.00036-17. PMID: 29118049; PMCID: PMC5706746.
https://pubmed.ncbi.nlm.nih.gov/29118049/ - Robertson RC, Manges AR, Finlay BB, Prendergast AJ. The Human Microbiome and Child Growth – First 1000 Days and Beyond. Trends Microbiol. 2019 Feb;27(2):131-147. doi: 10.1016/j.tim.2018.09.008. Epub 2018 Oct 24. PMID: 30529020.
https://pubmed.ncbi.nlm.nih.gov/30529020/ - Integrative HMP (iHMP) Research Network Consortium. The Integrative Human Microbiome Project. Nature. 2019 May;569(7758):641-648. doi: 10.1038/s41586-019-1238-8. Epub 2019 May 29. PMID: 31142853; PMCID: PMC6784865.
https://pubmed.ncbi.nlm.nih.gov/31142853/ - Dominguez-Bello MG, Godoy-Vitorino F, Knight R, Blaser MJ. Role of the microbiome in human development. Gut. 2019 Jun;68(6):1108-1114. doi: 10.1136/gutjnl-2018-317503. Epub 2019 Jan 22. PMID: 30670574; PMCID: PMC6580755.
https://pubmed.ncbi.nlm.nih.gov/30670574/ - Valdes AM, Walter J, Segal E, Spector TD. Role of the gut microbiota in nutrition and health. BMJ. 2018 Jun 13;361:k2179. doi: 10.1136/bmj.k2179. PMID: 29899036; PMCID: PMC6000740.
https://pubmed.ncbi.nlm.nih.gov/29899036/ - Kaan AMM, Kahharova D, Zaura E. Acquisition and establishment of the oral microbiota. Periodontol 2000. 2021 Jun;86(1):123-141. doi: 10.1111/prd.12366. Epub 2021 Mar 10. PMID: 33690935; PMCID: PMC8252790.
https://pubmed.ncbi.nlm.nih.gov/33690935/ - Wan Z, Zheng J, Zhu Z, Sang L, Zhu J, Luo S, Zhao Y, Wang R, Zhang Y, Hao K, Chen L, Du J, Kan J, He H. Intermediate role of gut microbiota in vitamin B nutrition and its influences on human health. Front Nutr. 2022 Dec 13;9:1031502. doi: 10.3389/fnut.2022.1031502. PMID: 36583209; PMCID: PMC9792504.
https://pubmed.ncbi.nlm.nih.gov/36583209/